Building the Zebrafish Lateral Line System
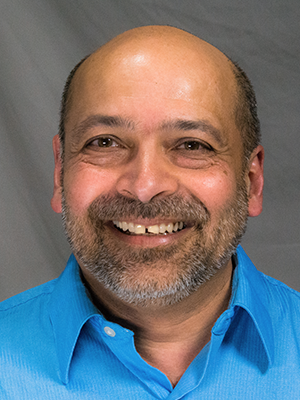
- Ajay Chitnis, MBBS, PhD, Head, Section on Neural Developmental Dynamics
- Damian E. Dalle Nogare, PhD, Staff Scientist
- Gregory Palardy, BS, Research Technician
- Chongmin Wang, MS, Research Technician
- Harsha Mahabaleshwar, PhD, Postdoctoral Fellow
- Uma Neelathi, PhD, Postdoctoral Fellow
- Caitlin Fox, BS, Graduate Student
- Marci Rosenberg, BS, Postbaccalaureate Intramural Research Training Award Fellow
Our current studies examine how the posterior lateral line system is built in the zebrafish nervous system. Our goal is to define the genetic regulatory network that coordinates cell fate and morphogenesis in the lateral line system and to build computational models that help us understand how this relatively simple and extremely accessible sensory system in zebrafish builds itself.
The lateral line is a mechanosensory system that detects water flow and consists of sensory organs called neuromasts, which are distributed in a stereotypic pattern over the surface of the zebrafish. Each neuromast has sensory hair cells at its center, which are surrounded by support cells that serve as progenitors for production of more hair cells during growth and regeneration of neuromasts. The development of this superficial sensory system in zebrafish can be easily observed in live embryos with transgenic lines expressing fluorescent proteins in specific subsets of cells of the lateral line system. In addition, a range of genetic and cellular manipulations can be used to investigate gene function.
The function of sensory hair cells in fish neuromasts is remarkably similar to that of hair cells in the vertebrate ear. Furthermore, the gene-regulatory network that determines specification of neuromast hair cells is very similar to the one specifying hair-cell fate in the human ear. Like the hair cells in our ears, neuromast hair cells can be damaged by exposure to drugs such as aminoglycosides, to copper ions, and to noise. However, unlike our ears, in which the loss of hair cells can be permanent, neuromast hair cells have a remarkable ability to regenerate. Hence, the lateral line system serves as an excellent model system for understanding development and for developing strategies to engineer regeneration of sensory hair cells.
The posterior lateral line system is initially established by the posterior lateral line (pLL) primordium, a cluster of about a 100 cells that migrate from the ear to the tip of the tail, periodically depositing neuromasts. Recent studies showed that the mechanisms that determine and guide collective migration and deposition of cells from the pLL primordium are remarkably similar to those that determine the collective migration of metastatic cancer cells. Hence, the lateral line system has also recently emerged as an excellent system for studying the biology of metastatic cancer cells.
Our expectation is that understanding the genetic regulatory network that coordinates cell fate in and morphogenesis of the zebrafish lateral line system will ultimately have a profound impact on translational studies that address a wide range of issues, including the development and regeneration of sensory systems and therapies directed at limiting the spread of cancer through metastasis.
Self-organization of the zebrafish lateral line primordium
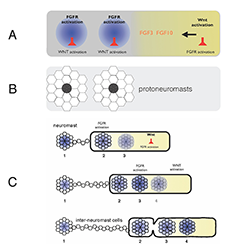
Click image to enlarge.
Self-organization of zebrafish lateral line primordium
A. FGFs secreted in response to Wnt activity in a leading zone (yellow) activate FGF receptors (FGFRs) in the trailing zone (blue). Wnt activity inhibits FGFR activation, and FGFR activation induces expression of a secreted factor that inhibits Wnt activity. B. Activation of FGFRs coordinates formation of protoneuromasts, in which cells reorganize to form epithelial rosettes, and a central cell (black) is specified as a sensory hair-cell precursor. C. Once about two neuromasts form, the primordium starts migrating. The Wnt zone (yellow) progressively shrinks as new protoneuromasts form progressively closer to the leading end. As the Wnt system shrinks, so does the primordium, and neuromasts and interneuromast cells are shed from the trailing end.
Interactions between the Wnt, FGF (fibroblast growth factor), Notch, BMP (bone morphogenetic protein), and chemokine signalling pathways in the pLL primordium provide a framework for understanding how cell fate and morphogenesis are coordinated in this group of about a hundred cells, as they collectively migrate under the skin from the ear to the tip of the tail. We used a combination of experiment and computational modeling to define the mechanisms that determine both the periodic formation of neuromasts and their deposition along with inter-neuromast cells, as the pLL primordium migrates along a path defined by chemokine expression.
Wnt proteins initially activate Wnt-beta catenin in a broad leading zone of the primordium. Cells with Wnt activity respond by becoming a source of FGF ligands. At the same time, they express factors that prevent a response to these FGFs. As leading cells with relatively high levels of Wnt activity are prevented from responding to the FGFs, an FGF–responsive center is initially established at the trailing end of the pLL primordium, where Wnt activity is weakest. The activation of FGF receptors in these trailing cells coordinates formation of nascent neuromasts, where cells reorganize to form epithelial rosettes, and a central cell is specified as a sensory hair-cell progenitor. However, the FGF–responsive center also becomes the source of a secreted Wnt antagonist, which progressively restricts the domain of active Wnt activity to the smaller leading zone. The shrinking Wnt system allows the formation of another FGF signaling–dependent 'protoneuromast' in its wake. In this manner, as the Wnt system shrinks, new protoneuromasts are formed, progressively closer to its leading end. After about the first two protoneuromasts form, the primordium starts migrating toward the tail.
As the pLL primordium migrates, cells are deposited from its trailing end, and the pLL primordium progressively shrinks. Cells that were incorporated into protoneuromasts are deposited as neuromasts, while cells that were not effectively incorporated into epithelial rosettes are deposited as interneuromast cells. Interestingly, shrinkage of the pLL primordium correlates with shrinking of the Wnt system, and the length of the Wnt active zone is always roughly 60% of the length of the primordium. While the reason for this correlation remains unclear at this time, knowing the initial size of the Wnt system, and the rate at which it shrinks provides a way to predict the permitted length of the primordium at any point during the course of migration. Trailing cells in a position that exceeds the permitted length of the primordium slow down, stop migrating, and are shed from the trailing end. The rate at which cells leave the migrating primordium depends on the rate at which the Wnt system shrinks and on the cell proliferation rate, as addition of cells adds to the length of the primordium, and hence to the possibility that trailing cells will fall outside the permitted length of the migrating primordium.
To build an agent-based model of lateral line primordium neuromast formation and deposition, we used data on the initial size of the primordium, the rate of Wnt shrinking, the average speed of the primordium, and the fact that the primordium stops migrating and terminates after the Wnt system reaches a critical minimum size. The model now provides a fairly good prediction of the average distance the primordium is expected to migrate and the average number neuromasts it is expected to deposit before it terminates. Though close, the model still slightly overestimates the number of neuromasts deposited and underestimates the distance travelled. Current studies are aimed at identifying deficits in our understanding that might be contributing to the discrepancies.
Publications
- Dalle Nogare D, Somers K, Rao S, Matsuda M, Reichman-Fried M, Raz E, Chitnis AB. Leading and trailing cells cooperate in collective migration of the zebrafish posterior lateral line primordium. Development 2014; 141(16):3188-3196.
Collaborators
- Hari Shroff, PhD, Laboratory of Molecular Imaging and Nanomedicine, NIBIB, Bethesda, MD
Contact
For more information, email chitnisa@mail.nih.gov.