Phosphoinositide Messengers in Cellular Signaling and Trafficking
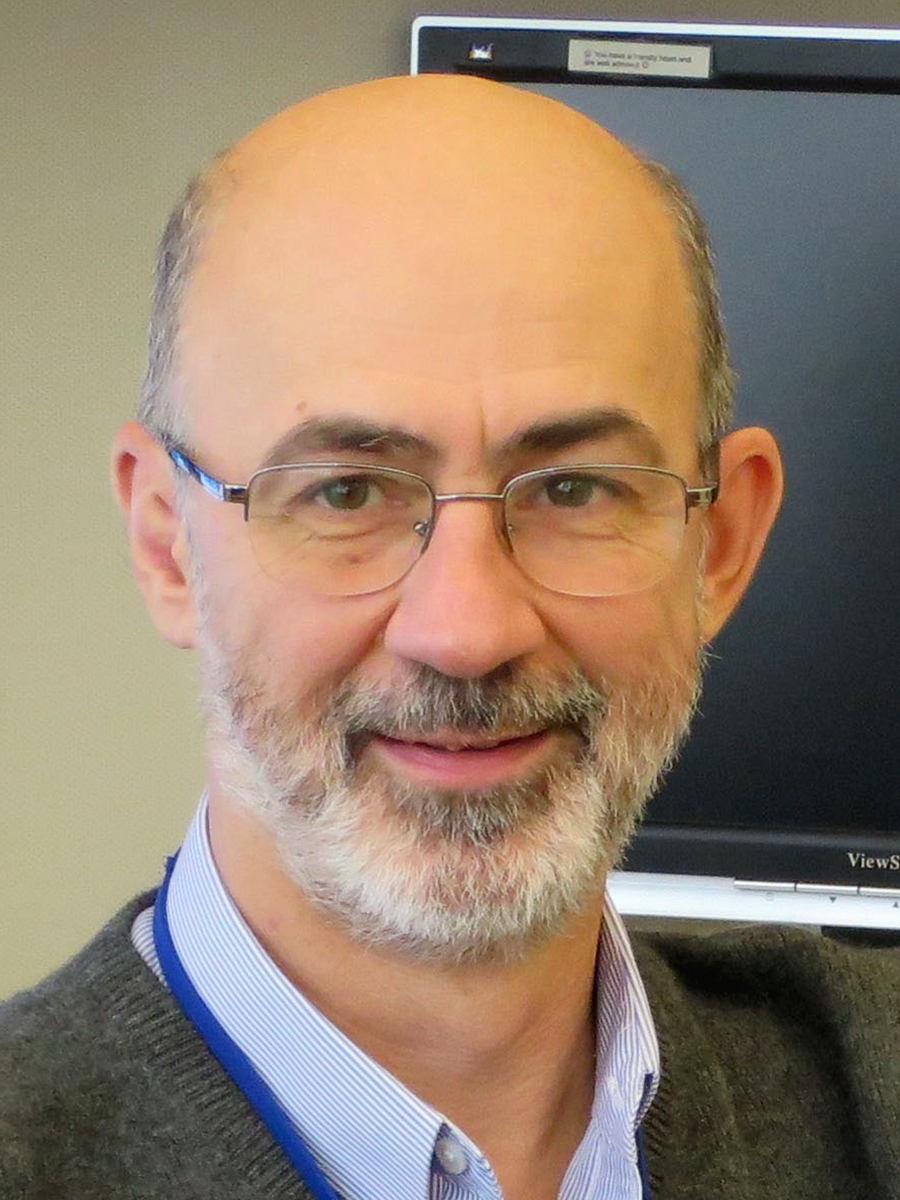
- Tamás Balla, MD, PhD, Head, Section on Molecular Signal Transduction
- Yeun Ju Kim, PhD, Staff Scientist
- Alejandro Alvarez-Prats, PhD, Postdoctoral Fellow
- Takashi Baba, PhD, Postdoctoral Fellow
- Joshua Pemberton, PhD, Postdoctoral Fellow
- Nivedita Sengupta, PhD, Postdoctoral Fellow
- Mira Sohn, PhD, Postdoctoral Fellow
- Dániel Tóth, MD, PhD, Postdoctoral Fellow
- Ljubisa Vitkovic, PhD, Special Volunteer
- Elisa Arthofer, MS, Graduate Student
We investigate signal transduction pathways that mediate the actions of hormones, growth factors, and neurotransmitters in mammalian cells, with special emphasis on the role of phosphoinositide-derived messengers. Phosphoinositides constitute a small fraction of the cellular phospholipids but play critical roles in the regulation of many signaling protein complexes, which assemble on the surface of cellular membranes and control a variety of cellular functions. Phosphoinositides regulate protein kinases and GTP–binding proteins as well as membrane transporters, including ion channels, thereby influencing many cellular processes, such as proliferation, apoptosis, metabolism, cell migration, and differentiation. We focus on the phosphatidylinositol 4 (PtdIns4)–kinases (PI4Ks), a family of enzymes that catalyze the first committed step in polyphosphoinositide synthesis. Current work aims to: (1) understand the function and regulation of several PI4Ks involved in the control of cellular signaling and trafficking pathways; (2) find specific inhibitors for the individual PI4Ks; (3) define the molecular basis of phosphatidylinositol 4-phosphate (PtdIns4P)–regulated pathways by identifying PtdIns4P–interacting molecules; (4) develop tools to analyze inositol lipid dynamics in live cells; and (5) determine the importance of the lipid-protein interactions in the activation of cellular responses by G protein–coupled receptors and receptor tyrosine kinases.
Molecular anatomy of the activation of the ER–resident Ca2+–regulatory protein STIM1
We studied the molecular events that control the entry of Ca2+ into mammalian cells. Ca2+ is one of the most important signaling molecules in the cell, and its concentration is kept at very low levels (about 10-7 M) in the cytoplasm. Cells elevate Ca2+ from this low level to trigger a variety of cellular pathways by releasing Ca2+ from intracellular storage organelles such as the endoplasmic reticulum (ER) or by opening specific gates at the plasma membrane (PM) for Ca2+ entry from outside the cell. An important Ca2+ entry pathway, called Store-Operated Calcium Entry (SOCE), consists of the PM Orai1 channels, which are activated by ER–localized STIM1 molecules that respond to depletion of the luminal Ca2+ content of the ER. Mutations in Orai1 and STIM1 that cause various diseases, most prominently severe immune deficiencies, have been detected in humans. Upon ER Ca2+ store depletion, STIM1 molecules cluster and activate the PM Orai1 channels via their SOAR (Stim1-Orai-Activation Region) domain. It is not well understood how the information on ER luminal Ca2+ decrease is transmitted to the cytoplasmic part of STIM1 through the single transmembrane segment. A key step in STIM1 activation is the release of its SOAR domain from an intramolecular clamp formed with the part of the STIM1 molecule that immediately follows the transmembrane region in the cytosolic side; this membrane-adjacent region is called the coiled-coil (CC1) region because of its predicted secondary structure. We used STIM1 molecules that were truncated immediately after the CC1 domain to show that they are capable of capturing or releasing the isolated the SOAR domain, depending on luminal ER Ca2+ concentrations. Using this limited STIM1 construct, we analyzed the very early molecular events that control the intramolecular clamp formed between the CC1 and SOAR domains. We found that STIM1 molecules form constitutive dimers even in the resting state and that their CC1 domain can also bind to the SOAR of another STIM1 molecule in trans. We developed a unique approach to oligomerize STIM1 molecules by targeting multiples of a small protein module, called FRB, to the ER lumen. These FRB multimers could oligomerize STIM1 molecules engineered by adding of a small protein module (called FKBP12) to their ER luminal side after adding rapamycin, which induces binding of FRBs to FKBP modules. Such artificial oligomerization, however, failed to liberate the SOAR domain or activate STIM1 molecules, whereas decreasing ER luminal Ca2+ was still capable of activation. The data suggested that oligomerization alone cannot trigger STIM1 activation. We propose that the release of SOAR from the STIM1–CC1 interaction is controlled by changes in the orientation of the two CC1 domains in STIM1 dimers upon Ca2+ unbinding. The importance of these studies is that they shed new light on the STIM1 activation process and could thus help us better understand how these molecules work and how the process is affected by mutations that cause human diseases due to SOCE dysfunction. The studies can also identify new ways to pharmacologically manipulate this process for the benefit of patients suffering from either hyperactivation or defective activation of the pathway.
The role of phosphatidylinositol 4-kinases during phagocytosis
A study performed in collaboration with the group of Sergio Grinstein made use of our recently developed tool to visualize phosphatidylinositol 4-phosphate (PI4P), a small regulatory lipid, in living phagocytic cells. Grinstein’s group has been studying the molecular events that govern the various steps during phagocytosis in macrophages. Phagocytosis is one of the most ancient defense mechanisms against foreign organisms (bacteria or cells), and it also plays critical roles in clearing cellular debris following cell death or tissue damage. Phosphoinositide lipids play an important role in all steps of phagocytosis, but changes in PI4P and their importance have not been explored because of the lack of appropriate tools to visualize the lipid during the process.
Using our recently developed PI4P reporter, the Grinstein group showed that PI4P undergoes a biphasic change during phagocytosis, a change that is localized to the phagocytic cup membrane during its maturation. PI4P, which is present in the plasma membrane (PM) before engagement of the target particle, is transiently enriched in the phagosomal cup. After the phagosome seals, i.e., it closes around the particle, PI4P levels rapidly drop as a result of the hydrolytic activity by Sac2, a PI4P phosphatase, and phospholipase C, the enzyme that hydrolyzes PI4P and PI(4,5)P2. PI4P disappearance coincides with the emergence of phagosomal PtdIns3P (PI3P), another inositol lipid that only differs from PI4P in the position of the phosphate on the inositol ring and which is formed by a different enzyme. Conversely, the disappearance of PI3P, which signals the transition from early to late phagosomes, is accompanied by resurgence of PI4P, which is associated with the recruitment of phosphatidylinositol 4-kinase 2A (PI4K2A). This secondary appearance of PI4P can be prevented by silencing PI4K2A or by eliminating PI4P by a recruitable form of Sac1, another PI4P 4-phosphatase, using the FRB–FKBP heterodimerization system (a system that is based on the rapamycin-induced heterodimerization of the FKBP12 protein and the small FKBP12–interaction region of the mTOR protein, called FRB). Importantly, the secondary accumulation of PI4P was found to be necessary for proper phagosomal acidification. The results demonstrated the complex dynamics of PI4P during phagocytosis and suggested that this phosphoinositide plays important roles during the maturation of the phagosome. The significance of these studies is that they highlighted a hitherto unrecognized and important role of PI4P in phagocytosis. A better understanding of the molecular players in the phagocytic process will enhance our ability to counter the processes that parasitic bacteria developed to evade degradation by lysosomes.
The role of inositol lipids in membrane recruitment of Ras proteins
Ras proteins are small globular proteins functioning as molecular switches that change conformation upon GTP binding, a conformation that is distinct from from the GDP–bound forms. Ras protein mutations are some of the most common causes in cancer and hence have attracted much attention. Membrane attachment of Ras proteins is critical to their signaling function, and it is their C-termini that contain localization signals providing for membrane attachment. Of the three forms of Ras proteins (N-, H-, and K-Ras), K-Ras has a polybasic sequence preceding its C-terminal farnesyl lipid modification. In contrast, N-Ras has a palmitoyl residue in addition to the C-terminal prenyl modification. It has been known that the polybasic residue of K-Ras is critical for its membrane interaction via binding to phosphoinositides. In collaboration with Péter Várnai’s group, we investigated how the rapid depletion of PM PPIns can regulate the intracellular distribution of the K- and H-Ras proteins. Várnai’s group created a BRET sensor for monitoring the movement of PM–bound Ras proteins and followed them after they are released from the PM. The analysis showed that K-Ras or its isolated targeting sequence moves to either the ER or the Golgi upon the rapid depletion of PM phosphoinositides (PPIns) caused by receptor stimulation. No similar translocation was found with the N-Ras proteins. Furthermore, PM PPIns depletion significantly attenuated the proliferation of cells expressing constitutively active K- but not H-Ras mutants, highlighting the different functional role of the PPIns–dependent PM anchoring in K-Ras and H-Ras signaling. The importance of these studies is that they help us understand the molecular determinants of Ras–membrane interactions and that they showed that Golgi-associated K-Ras proteins are unable to elicit a full proliferative effect.
Additional Funding
- NSERC Postdoctoral Fellowship supporting Dr. Joshua Pemberton
- JSPS-NIH Fellowship supporting Dr. Takashi Baba
Publications
- Sohn M, Ivanova P, Brown HA, Toth DJ, Varnai P, Kim YJ, Balla T. Lenz-Majewski mutations in PTDSS1 affect phosphatidylinositol 4-phosphate metabolism at ER-PM and ER-Golgi junctions. Proc Natl Acad Sci USA 2016 113:4314-4319.
- Korzeniowski MK, Wisniewski E, Baird B, Holowka DA, Balla T. Molecular anatomy of the early events in STIM1 activation—oligomerization or conformational change? J Cell Sci 2017 130:2821-2832.
- Levin R, Hammond GR, Balla T, De Camilli P, Fairn GD, Grinstein S. Multiphasic dynamics of phosphatidylinositol 4-phosphate during phagocytosis. Mol Biol Cell 2017 28:128-140.
- Gulyás G, Radvánszki G, Matuska R, Balla A, Hunyady L, Balla T, Várnai P. Plasma membrane phosphatidylinositol 4-phosphate and 4,5-bisphosphate determine the distribution and function of K-Ras4B but not H-Ras proteins. J Biol Chem 2017; ePub ahead of print.
- Várnai P, Gulyás G, Tóth DJ, Sohn M, Sengupta N, Balla T. Quantifying lipid changes in various membrane compartments using lipid binding protein domains. Cell Calcium 2017 64:72-82.
Collaborators
- Barbara Baird, PhD, Cornell University, Ithaca, NY
- Evžen Boura, PhD, Institute of Organic Chemistry and Biochemistry, Academy of Sciences of the Czech Republic, Prague, Czech Republic
- Sergio Grinstein, PhD, Hospital For Sick Children, Toronto, Canada
- David Holowka, PhD, Cornell University, Ithaca, NY
- Péter Várnai, MD, PhD, Semmelweis University, Faculty of Medicine, Budapest, Hungary
Contact
For more information, email ballat@mail.nih.gov or visit https://irp.nih.gov/pi/tamas-balla.