Interplay between Membrane Organelles, Cytoskeleton, and Metabolism in Cell Organization and Function

- Jennifer Lippincott-Schwartz, PhD, Head, Section on Organelle Biology
- Chad D. Williamson, PhD, Research Fellow
- Dani Cai, PhD, Visiting Fellow
- Sarah Cohen, PhD, Visiting Fellow
- Yang Niu, PhD, Visiting Fellow
- Alex Valm, PhD, Postdoctoral Fellow
- Lingfeng Chen, PhD, Volunteer
- Christopher Obara, PhD, Postdoctoral Fellow
- Carolyn Ott, PhD, Staff Scientist
- Prabuddha Sengupta, PhD, Postdoctoral Fellow
- Arnold Seo, PhD, Postdoctoral Fellow
We investigate the global principles underlying cell behavior at both small and large spatial scales. At the small scale, we employ the super-resolution imaging techniques of photoactivated localization microscopy (PALM), interferometric 3D PALM, single-particle tracking PALM, and pair-correlation PALM to map the spatial organization, stoichiometry, and dynamics of proteins associated with various membrane-bound compartments and with the cytoskeleton. We also employ fluorescence photobleaching, photoactivation, fluorescence correlation, and fluorescence energy transfer methods to measure protein-protein interactions, protein turnover rates, and protein association rates. Such approaches allow us to assay cellular functions, including receptor stoichiometry and protein clustering and diffusion behavior at the nanometric scale in living cells. At the large scale, we investigate how complex behaviors of cells arise, such as cell crawling, polarization, cytokinesis, and viral budding. We study these complex behaviors by quantitatively analyzing diverse intracellular processes, including membrane trafficking, autophagy, actin/microtubule dynamics, and organelle assembly/disassembly pathways, which undergo dramatic changes as cells alter their behavior and organization throughout life. To support these efforts, we combine various fluorescence-based imaging approaches, including total internal reflection fluorescence (TIRF) microscopy imaging and spinning-disk and laser-scanning confocal microscopy, with FRAP (fluorescence recovery after photobleaching), FLIP (fluorescence loss in photobleaching), and photoactivation to obtain large image data sets. We process the data sets computationally to extract biochemical and biophysical parameters, which can be related to the results of conventional biochemical assays. We then use the results to generate mechanistic understanding and predictive models of the behavior of cells and subcellular structures (including endoplasmic reticulum, Golgi, cilia, endosomes, lysosomes, autophagosomes, and mitochondria) under healthy and pathological conditions.
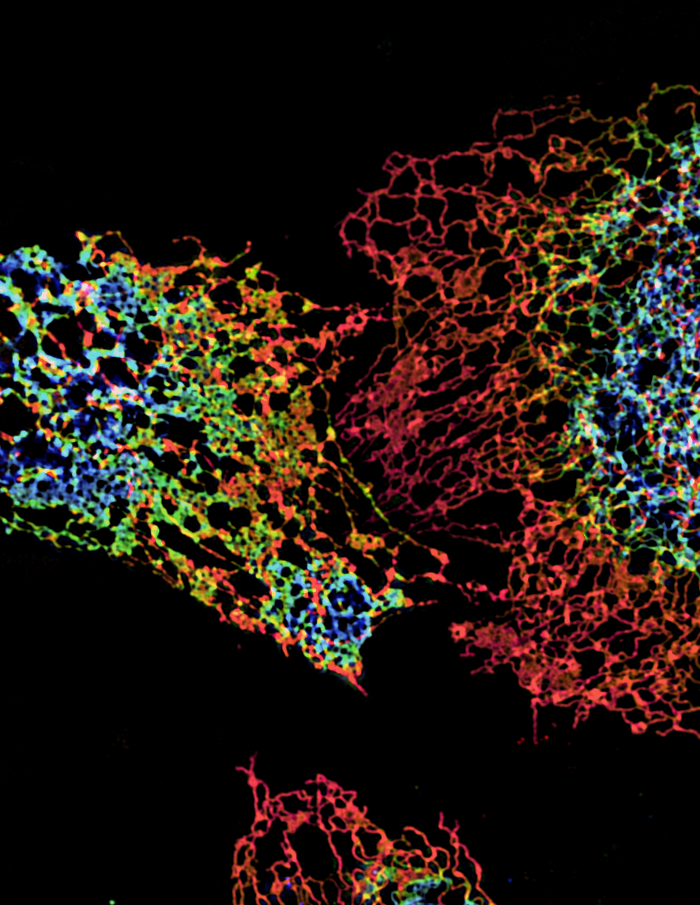
Click image to enlarge.
ER structure visualized using structured illumination microscopy (SIM)
Structure of peripheral endoplasmic reticulum (ER) in three cells labeled with an ER protein marker visualized by three-dimensional SIM. The color coding represents different z heights of the ER.
ER structure and dynamics visualized with increased spatio-temporal resolution
The endoplasmic reticulum (ER) consists of interconnected tubules and flattened sheets that extend from the nuclear envelope to the periphery of the cell, impacting every cellular compartment through its contacts and functional interactions. Mutations in proteins that regulate the shape of the ER lead to various neurological disorders. We employed five different super-resolution technologies with complementary strengths and weaknesses in spatial and temporal capabilities to study the fine morphology and dynamics of the peripheral ER. A high-speed variation of structured illumination microscopy (SIM) allowed ER dynamics to be visualized at unprecedented speeds and resolution. Three-dimensional SIM (3D-SIM) and Airyscan imaging allowed comparison of the fine distributions of different ER–shaping proteins. Lattice light sheet point accumulation for imaging in nanoscale topography (LLS-PAINT) and focused ion-beam scanning electron microscopy (FIB-SEM) permitted 3D characterization of various ER structures. Using these approaches, we observed that many ER structures previously proposed to be flat membrane sheets are instead densely packed tubular arrays, which we call ER matrices. The matrices were extremely compact, with spaces between tubules below the resolving power of most super-resolution methodologies. We also discovered that ER tubules and junctions undergo fast oscillations, rapidly interconverting from tight to loose arrays. The oscillations of tubules and junctions were energy-dependent and allowed the ER to interconvert between tight and loose tubule networks. Our finding of dense tubular matrices in areas previously thought of as flat sheets provides a new model for maintaining and generating ER structure. In this model, ER matrices would sequester excess membrane proteins and lipids, and their dynamic interconversion into loose tubule arrays would permit the ER to rapidly extend its shape to reach the cell periphery, for example during cell locomotion.
ER trapping reveals that Golgi enzymes continually revisit the ER through a recycling pathway that controls Golgi organization.
The Golgi apparatus is the major processing and sorting station at the crossroads of the secretory pathway, containing specialized sorting and transport machinery, which either drive secretory trafficking or filter out selected membrane and protein components for return to the ER for continued use. How the Golgi maintains its structure and function amidst this ongoing bi-directional membrane trafficking has been the subject of a long-standing debate. In particular, it is unclear whether Golgi enzymes remain localized within the Golgi or constitutively cycle through the ER.
To address this question, we used a rapamycin trapping assay to test whether Golgi enzymes become trapped in the ER upon expression of both an ER–trapping protein and Golgi enzyme bait. We found that, within four hours of rapamycin treatment, Ii-FKBP12 (i.e., the ER trapper) trapped nearly all Golgi-localized FKBP-rapamycin binding domain (FRB)–Golgi enzyme (i.e., the Golgi bait) in the ER. Direct redistribution from the Golgi to the ER during rapamycin treatment occurred because selective photoactivation of the FRB–tagged, photoactivatable Golgi enzyme in the Golgi resulted in the signal shifting to the ER. In the ER, fluorescent forms of FRB–Golgi enzyme and Ii-FKBP12 underwent FRET (fluorescence energy transfer), indicating direct binding upon rapamycin-induced redistribution. By contrast, use of Ii-FRB, which we showed is an inefficient ER trap, resulted in minimal redistribution of FKBP12–tagged Golgi enzymes during rapamycin treatment. These data demonstrated that Golgi enzymes constitutively cycle through the ER. Using our trapping scheme, we further identified roles of the protein Rab6a and the phospholipase iPLA 2 in Golgi enzyme recycling and showed that retrograde transport of Golgi membrane is necessary for Golgi dispersal during microtubule depolymerization and mitosis.
Publications
- Valm AM, Cohen S, Legant WR, Melunis J, Hershberg U, Wait E, Cohen AR, Davidson MW, Betzig E, Lippincott-Schwartz J. Applying systems-level spectral imaging and analysis to reveal the organelle interactome. Nature 2017 546(7656):162-167.
- Ritter AT, Kapnick SM, Murugesan S, Schwartzberg PL, Griffiths GM, Lippincott-Schwartz J. Cortical actin recovery at the immunological synapse leads to termination of lytic granule secretion in cytotoxic T lymphocytes. Proc Natl Acad Sci 2017 114(32):E6585-E6594.
- Waxse BJ, Sengupta P, Hesketh GG, Lippincott-Schwartz J, Buss F. Myosin VI facilitates connexin 43 gap junction accretion. J Cell Sci 2017 130(5):827-840.
- Yao PJ, Manor U, Petralia RS, Brose RD, Wu RT, Ott C, Wang YX, Charnoff A, Lippincott-Schwartz J, Mattson MP. Sonic hedgehog pathway activation increases mitochondrial abundance and activity in hippocampal neurons. Mol Biol Cell 2017 28(3):387-395.
- Nixon-Abell J, Obara CJ, Weigel AV, Li D, Legant WR, Xu CS, Pasolli HA, Harvey K, Hess HF, Betzig E, Blackstone C, Lippincott-Schwartz J. Increased spatiotemporal resolution reveals highly dynamic dense tubular matrices in the peripheral ER. Science 2016 354(6311):aaf3928.
Collaborators
- Eric Betzig, PhD, Howard Hughes Medical Institute, Janelia Farm Research Campus, Ashburn, VA
- Craig Blackstone, MD, PhD, Cell Biology Section, NINDS, Bethesda, MD
- Harald Hess, PhD, Howard Hughes Medical Institute, Janelia Farm Research Campus, Ashburn, VA
- Uri Manor, PhD, Salk Institute for Biological Studies, La Jolla, CA
- Mark P. Matteson, PhD, Laboratory of Neurosciences, NIA, Baltimore, MD
- Jonathon J. Nixon-Abell, PhD, Cell Biology Section, NINDS, Bethesda, MD
- George Patterson, PhD, Section on Biophotonics, NIBIB, Bethesda, MD
- Alex T. Ritter, PhD, Genentech, San Francisco, CA
- Bennett Waxse, MD, PhD, University of Chicago Medicine, Chicago, IL
- Pamela J. Yao, PhD, Cellular and Molecular Neurosciences Section, NIA, Baltimore, MD
Contact
For more information, email lippincj@mail.nih.gov or visit http://lippincottschwartzlab.nichd.nih.gov.