Extracellular Matrix Disorders: Molecular Mechanisms and Treatment Targets
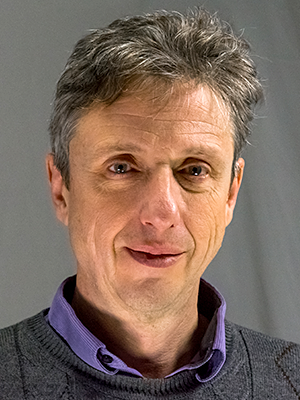
- Sergey Leikin, PhD, Head, Section on Physical Biochemistry
- Elena N. Makareeva, PhD, Staff Scientist
- Edward L. Mertz, PhD, Staff Scientist
- Shakib Omari, PhD, Postdoctoral Fellow
- Anna Roberts-Pilgrim, PhD, Postdoctoral Fellow
- Laura Gorrell, BS, Predoctoral Fellow
The extracellular matrix (ECM) is involved in a wide variety of disorders, ranging from rare genetic abnormalities of skeletal development (skeletal dysplasias) to such common ailments as osteoporosis, fibrosis, and cancer. Our interest in ECM biology began with studies on basic principles relating the helical structure of collagen and DNA to their interactions and biological function. Over the years, the focus of our research shifted to collagens, which are the most abundant ECM molecules, and then to ECM disorders and the development of novel treatments for these disorders. We gradually phased out DNA studies and concentrated on ECM pathology in cancer, fibrosis, osteogenesis imperfecta (OI), Ehlers-Danlos syndrome (EDS), chondrodysplasias, osteoporosis, and other diseases. Together with other NICHD and extramural clinical scientists, we strive to improve our knowledge of the molecular mechanisms underlying those diseases. We hope to use the knowledge gained through our studies for diagnostics, characterization, and treatment, bringing our expertise in physical biochemistry and theory to clinical research and practice.
Procollagen folding and its role in bone disorders
Collagens are triple-helical proteins forming structural scaffolds of many tissues and organs. Type I is the most common collagen. It is by far the most abundant protein in all vertebrates and the main structural protein of extracellular matrix (ECM) in bone, skin, and other tissues. Its procollagen precursor is assembled from two pro-alpha1(I) and one pro-alpha2(I) chains, folded in the endoplasmic reticulum (ER), trafficked through the Golgi apparatus, secreted, and then converted into a 300-nm-long triple helix of mature collagen by cleavage of C- and N-propeptides. Osteoblasts (cells responsible for making bone) produce and secrete the massive amounts of type I procollagen needed to build the skeleton, presenting a unique challenge for protein quality control and trafficking. Not only does an osteoblast secrete enough procollagen to fill an entire cell volume in just a day, but procollagen is one of the most difficult proteins to fold. We discovered that the conformation of natively folded human procollagen is less thermodynamically favorable than the unfolded one above 35°C. To fold procollagen at body temperature, cells use specialized ER chaperones to stabilize the native conformation. Outside the cell, the native conformation is stabilized after procollagen is converted to collagen and incorporated into collagen fibrils. Unincorporated molecules denature within several hours of secretion and become susceptible to rapid proteolytic degradation. Up to 10–5% of procollagen is misfolded even under normal conditions, necessitating activation of cell stress–response pathways responsible for degradation of misfolded molecules and forcing an active osteoblast to always function in a high-stress mode. Our findings indicate that one of key factors in bone pathology is osteoblast malfunction resulting from excessive cell stress, which is often caused by increased procollagen misfolding, inability of the cell to handle the normal load of misfolded procollagen, or both (Reference 1).
The most common hereditary cause of increased procollagen misfolding is a Gly substitution anywhere in the obligatory (Gly-X-Y)n sequence that distinguishes all collagens. Such substitutions in type I collagen are responsible for over 80% of severe OI cases. Similar substitutions in other collagens cause EDS and a variety of other syndromes. Our studies of OI patients with over 50 different Gly substitutions revealed several structural regions within the collagen where these mutations might be responsible for distinct OI phenotypes. For example, the first 85–90 amino acids at the N-terminal end of the triple helix form an “N-anchor” domain, mutations within which prevent normal N-propeptide cleavage. Incorporation of molecules with uncleaved N-propeptides into collagen fibrils leads to hyperextensibility and joint laxity more characteristic of EDS.
Bone pathology associated with excessive procollagen misfolding of nonhereditary origin is likely to be more prevalent than OI. Indeed, our data suggest that such misfolding should occur upon changes in the osteoblast ER environment associated with aging, environmental factors, inflammation, etc. It is likely to contribute to age-related osteoporosis, bone loss during cancer treatment, and many other common ailments. However, almost nothing is known about this pathophysiology mechanism because procollagen folding and consequences of its misfolding for the cell remain poorly understood.
Cell biology of procollagen misfolding
Based on all these findings, our current research is focused primarily on the cell biology of procollagen misfolding. In one approach, we are collaborating with Jennifer Lippincott-Schwartz on using live-cell imaging to investigate the synthesis, folding, trafficking, and degradation of fluorescently tagged procollagen in osteoblasts. Live-cell imaging of osteoblasts transiently transfected with fluorescent procollagen chains revealed completely unexpected features of procollagen quality control and trafficking. We observed sorting of normally folded and misfolded procollagen molecules at COPII (a coatomer protein, i.e., a vesicle coat protein that transports proteins from the rough ER to the Golgi apparatus) ER exit sites (ERES). Normally folded procollagen was loaded into giant (up to 500 nm) Golgi-bound transport vesicles. Contrary to widely held beliefs, the vesicles did not have a COPII coat and did not contain HSP47, which is a collagen-specific ER chaperone that preferentially binds to natively folded procollagen to assist in its folding and loading into ERES. Apparently HSP47 was removed from procollagen upon its entry into ERES. Misfolded procollagen was retained at ERES, resulting in a COPII–dependent modification of ERES membrane by autophagic machinery and subsequent lysosomal engulfment and degradation. The findings delineate a novel, COPII–dependent, non-conventional micro-autophagy-like pathway for recycling ERES–loaded cargo.
To validate their physiological significance and further build on these findings, we are expanding our tool chest by exploiting emerging gene editing technologies. We have already created an osteoblast cell line in which endogenous proα2(I) is fluorescently tagged and which contains Flp–recombinase target sites for manipulating the tag, e.g., changing the fluorescence color or completely replacing it. Presently, we are introducing additional Flp-recombinase target sites into the same gene in order to manipulate almost the entire gene sequence. The strategy will enable us to perform live-cell imaging of endogenous rather than transiently transfected procollagen with a variety of OI mutations. It can then be used to generate mouse models and to study other proteins. Importantly, the discoveries we have already made may have wide implications beyond procollagen and ECM biology. For instance, COPII coat involvement in regulating autophagic degradation as well as cargo rerouting from the secretory to degradative pathway at ERES are likely to be general rather than collagen-specific phenomena. The hypothesis is currently under investigation in our and several collaborating laboratories. From clinical and translational perspectives, our findings may explain at least some of the pathology in patients with COPII mutations by deficient autophagic degradation of difficult-to-fold proteins, which is another line of investigation we and our collaborators hope to pursue in the future.
In another approach, we are investigating cell-stress response to procollagen with a Gly610 to Cys substitution in the triple helical region of proα2(I) in a mouse model of OI. The G610C mouse model mimics the Gly610 to Cys mutation found in a large group of patients from an Old Order Amish community in Pennsylvania. Our study of cultured fibroblasts and osteoblasts as well as of tissues in this model revealed misfolding and accumulation of mutant molecules in the ER. Elevated phosphorylation of the translation initiation factor EIF2a indicated the presence of cell stress, although we found no evidence of conventional unfolded-protein-response (UPR) signaling. We found that misfolded procollagen molecules are degraded by lysosomes via autophagy rather than by proteasomes via ER–associated degradation. Osteoblasts adapt to procollagen misfolding by enhancing autophagy and thereby reducing excessive accumulation of misfolded procollagen in the ER. Such an adaptation prevents cell death but is not sufficient to prevent abnormal cell function. The osteoblasts produce less and lower-quality bone matrix. Their abnormal differentiation into osteocytes appears to affect matrix mineralization as well. To compensate for reduced bone synthesis by each cell, G610C mice generate more osteoblasts. However, the combination of the resulting increase in bone formation surfaces with reduced bone formation rate at these surfaces disrupts normal bone modeling, causing, e.g., long-term entrapment of poorly organized woven bone between layers of lamellar bone. The disruption of the cortical bone matrix structure leads to more brittle bones, which have an increased susceptibility to fracture upon high energy impact despite normal cortical thickness and slightly higher cortical bone mineral density. (References 1, 2)
Development of novel OI treatments
We hypothesize that bone pathology associated with procollagen misfolding may be at least partially reversed by targeting the cell stress response to misfolded procollagen accumulation in the ER, thereby improving osteoblast function. Although this would not eliminate potentially detrimental effects of secreted mutant collagen in OI bone, pharmacological treatment of osteoblast malfunction is a more realistic short-term approach to OI than suppression of dominant negative OI mutations by gene therapy or bone marrow transplantation. Moreover, the same approach is likely to be a better long-term strategy for treatment of osteoblast malfunction caused by procollagen misfolding in cases that do not involve pathogenic mutations.
In pursuing this strategy, we are currently targeting procollagen autophagy. Provided that misfolded procollagen accumulation in the ER is indeed involved in osteoblast malfunction, the simplest way to prevent such accumulation is to enhance the natural ability of the cell to remove and degrade the misfolded molecules via autophagy. Our preliminary study of a low-protein diet's (LPD) effect on G610C mice provided encouraging evidence of improved osteoblast function as well as bone matrix quality and mineralization. However, in addition to enhancing autophagy, a LPD also causes cell stress associated with nutrient deprivation and alters animal growth. The latter and other confounding effects made the LPD study difficult to interpret (Reference 2). We are considering an intermittent LPD as a potential component of the eventual treatment strategy, but it may still have a variety of unintended consequences. To unequivocally validate autophagy as a target before embarking on optimizing dietary and pharmacological treatments, we are currently pursuing an approach based on altering the expression of Atg5, a key gene involved in the regulation of autophagosome formation.
Our experiments confirmed that reduced autophagy increases OI severity. In heterozygous G610C animals, with reduced Atg5 expression in all tissues, we observed close to 50% perinatal lethality compared with wild-type littermates, in contrast to negligible/undetectable perinatal lethality of heterozygous G610C mice with normal Atg5 expression. Conditional Atg5 knockout in mature osteoblasts resulted in dramatically increased bone malformations. Preliminary experiments pointed to even more severe bone defects associated with conditional Atg5 knockout in osteoblast precursors. To complete the study, we are presently examining Atg5 gain-of-function effects, e.g., by switching from low to normal/high Atg5 expression and by conditional targeted Atg5 overexpression.
Translational studies on patients with novel or unusual OI and EDS mutations
Abnormal collagen biosynthesis and malfunction of osteoblasts are also important factors in OI that is caused by other collagen mutations as well as by mutations in other proteins. Over the past several years, we assisted several clinical research groups in characterizing collagen biosynthesis and folding in fibroblasts from patients with newly discovered recessive forms of OI and closely related skeletal dysplasias caused by mutations in cartilage-associated protein (CRTAP), prolyl-3-hydrohylase (P3H1), cyclophilin B (CYPB), the collagen-binding molecular chaperone FKBP65, the signaling protein WNT1, the ER–membrane ion channel TRICB, Golgi-membrane metalloprotease S2P, and the transmembrane anterior posterior transformation protein 1 (TAPT1). In particular, our collaboration with Joan Marini suggested that the CRTAP/P3H1/CYPB complex functions as a procollagen chaperone. A deficiency in any of the three proteins delays procollagen folding, although their exact role in procollagen folding remains unclear. More surprisingly, we found no detectable changes in the procollagen folding rate in cultured fibroblasts from patients with FKBP65 mutations. Our data suggest that FKBP65 may affect post-translational modification of procollagen and deposition of collagen matrix by a different mechanism. It remains unclear why some FKBP65 mutations cause severe OI with joint contractures (Bruck syndrome) while others cause joint contractures without pronounced OI (Kuskokwim syndrome) or OI without pronounced joint contractures. Our study of TRICB–deficient cells revealed abnormal conformation and reduced thermal stability of type I procollagen, suggesting dysregulation of collagen chaperones in the ER or direct involvement of TRICB in procollagen folding. Our experiments indicated that pathogenic effects of mutations in the transmembrane protein TAPT1 and in site-2 metalloprotease (S2P) might not be directly related to disruptions in synthesis, folding, or trafficking of procollagen chains (Reference 3).
More recently, in collaboration with Carsten Bonnemann, we investigated collagen biosynthesis abnormalities caused by mutations in collagen prolyl-4-hydroxylase 1 (P4H1), which result in complex developmental abnormalities involving bones and other connective tissues (Reference 4). As expected, we found that patient skin fibroblasts secreted procollagen with significantly reduced thermal stability. However, we found no abnormalities in the procollagen folding or secretion rates and no evidence of misfolded procollagen accumulation in the cell. The latter findings are extremely surprising given that mutant P4H1 results in significantly reduced proline 4-hydroxylation, which is believed to be important for procollagen triple helix folding. We hypothesize that the folding rate is normalized through some compensatory action of procollagen chaperones. Consistently, our ongoing experiments point to abnormal composition of ER chaperones, but much remains to be done before we understand how patient fibroblasts manage to fold and secrete a normal amount of procollagen despite the P4H1 deficiency and how this deficiency causes connective tissue pathology.
Extracellular matrix pathology in tumors and fibrosis
Another important advance from our work of the past several years was the characterization of a collagenase-resistant, homotrimeric isoform of type I collagen and its potential role in cancer, fibrosis, and other disorders. The normal isoform of type I collagen is a heterotrimer of two alpha1(I) chains and one alpha2(I) chain. Homotrimers of three alpha1(I) chains are produced in some fetal tissues, carcinomas, fibrotic tissues, as well as in rare forms of OI and EDS associated with alpha2(I) chain deficiency. We found the homotrimers to be at least 5–10 times more resistant to cleavage by all mammalian collagenases than the heterotrimers, and we determined the molecular mechanism of this resistance. Our studies suggested that cancer cells might utilize the collagen isoform to build collagenase-resistant tracks, thus supporting invasion through stroma of lower resistance.
We also collaborated with Constantine Stratakis' lab to investigate bone tumors caused by defects in protein kinase A (PKA), a key enzyme in the cAMP signaling pathway. Initially, we investigated synthesis of type I collagen homotrimers. However, over the last 3–5 years, the focus of the study has shifted to abnormal differentiation of osteoblastic cells and deposition of bone within these tumors. We found that knockouts of various PKA subunits cause not only abnormal organization and mineralization of bone matrix but also novel bone structures that had not been previously reported. For instance, we observed free-standing cylindrical bone spicules with an osteon-like organization of lamellae and osteocytes but an inverted mineralization pattern, a highly mineralized central core, and decreasing mineralization away from the central core. Currently, we are assisting the Stratakis lab in characterizing abnormal osteoblast maturation, the role of an abnormal inflammatory response, and effects of anti-inflammatory drug treatments in these animals (Reference 5). Improved understanding of bone tumors caused by PKA deficiencies may not only clarify the role of cAMP signaling but also suggest new approaches to therapeutic manipulation of bone formation in skeletal dysplasias.
Multi-modal micro-spectroscopic imaging and mapping of tissues
Label-free micro-spectroscopic infrared and Raman imaging of tissues and cell cultures provides important information about the chemical composition, organization, and biological reactions inaccessible by traditional histology. However, applications of these techniques were severely restricted by light-path instabilities in thin hydrated specimens under physiological conditions. We resolved the problem by designing specimen chambers with precise thermo-mechanical stabilization for high-definition (HD) infrared imaging and Raman micro-spectroscopy, achieving spectral reproducibility up to two orders of magnitude better than with leading commercial instruments. The HD technology was essential for the analysis of abnormal collagen matrix deposition by CRTAP– and FKBP65–deficient cells. It has enabled us to assist NIBIB scientists in characterizing a functionalized carbon-nanotube approach to the delivery of anticancer agents into cells that overexpress hyaluronate receptors and is crucial for our current studies of bone structure and mineralization in the mouse models of OI and PKA deficiencies described above.
The power of the technology is best illustrated by our studies of ECM structure and of composition effects on the function of cartilage in a mouse model of diastrophic dysplasia (DTD), an autosomal recessive dysplasia that affects cartilage and bone development and is caused by mutations in the SLC26A2 sulfate transporter, deficient sulfate uptake by chondrocytes, and resulting under-sulfation of glycosaminoglycans in cartilage matrix. In collaboration with Antonella Forlino and Antonio Rossi, we found that the deficiency results in under-sulfation of chondroitin and disorientation of collagen fibers, disrupting a thin protective layer at the articular surface and causing subsequent cartilage degradation. We investigated the relationship between chondroitin under-sulfation and the rate of its synthesis across the growing epiphyseal cartilage, and we built a mathematical model for the sulfation pathway, predicting treatment targets for sulfation-related chondrodysplasias and genes that might contribute to the juvenile idiopathic arthritis recently associated with single-nucleotide polymorphisms in the gene encoding the SLC26A2 transporter.
We are extending the technology by combining imaging of bone and cartilage ECM composition and structure with biomechanical measurements at the same length scales. The mechanical properties of bone and cartilage should depend on the deformation length scale because of the heterogeneous microscopic structure and the presence of different macroscopic regions and zones in these tissues. Nevertheless, biomechanical studies are rarely accompanied by mapping of tissue composition and structure. To address the problem, we are collaborating with Peter Basser and Emilios Dimitriadis on mapping cartilage elasticity by force microscopy at length scales appropriate for examining the material properties of the ECM and on combining it with our multimodal imaging technology.
While the ECM plays a key role in normal development and pathology of all tissues, most studies focus on expression of its components rather than its overall organization. Our multimodal imaging technology is helping to close this gap in in vitro studies of tissue sections and cell cultures. To translate these advances into clinical practice, we established a new collaboration with Basser on using the technology to calibrate and test newer methods for noninvasive in vivo ECM studies by the solid-state magnetic resonance imaging (MRI) that is being developed in his laboratory.
Publications
- Mirigian LS, Makareeva E, Mertz EL, Omari S, Roberts-Pilgrim AM, Oestreich AK, Phillips CL, Leikin S. Osteoblast malfunction caused by cell stress response to procollagen misfolding in alpha2(I)-G610C mouse model of osteogenesis imperfecta. J Bone Miner Res 2016 31:1608-1616.
- Mertz EL, Makareeva E, Mirigian LS, Koon KY, Perosky JE, Kozloff KM, Leikin S. Makings of a brittle bone: unexpected lessons from a low protein diet study of a mouse OI model. Matrix Biol 2016 52-54:29-42.
- Lindert U, Cabral WA, Ausavarat S, Tongkobpetch S, Ludin K, Barnes AM, Yeetong P, Weis M, Krabichler B, Srichomthong C, Makareeva EN, Janecke AR, Leikin S, Rothlisberger B, Rohrbach M, Kennerknecht I, Eyre DR, Suphapeetiporn K, Giunta C, Marini JC, Shotelersuk V. MBTPS2 mutations cause defective regulated intramembrane proteolysis in X-linked osteogenesis imperfecta. Nat Commun 2016 7:11920.
- Zou Y, Donkervoort S, Salo AM, Foley AR, Barnes AM, Hu Y, Makareeva E, Leach ME, Mohassel P, Dastgir J, Deardorff MA, Cohn RD, DiNonno WO, Malfait F, Lek M, Leikin S, Marini JC, Myllyharju J, Bonnemann CG. P4HA1 mutations cause a unique congenital disorder of connective tissue involving tendon, bone, muscle and the eye. Hum Mol Genet 2017 26:2207-2217.
- Saloustros E, Liu S, Mertz EL, Bhattacharyya N, Starost MF, Salpea P, Nesterova M, Collins M, Leikin S, Stratakis CA. Celecoxib treatment of fibrous dysplasia (FD) in a human FD cell line and FD-like lesions in mice with protein kinase A (PKA) defects. Mol Cell Endocrinol 2017 439:165-174.
Collaborators
- Peter Basser, PhD, Section on Quantitative Imaging and Tissue Sciences, NICHD, Bethesda, MD
- Carsten Bonnemann, MD, Neuromuscular and Neurogenetic Disorders of Childhood Section, NINDS, Bethesda, MD
- Peter H. Byers, MD, University of Washington, Seattle, WA
- Paul J. Coucke, PhD, Universitair Ziekenhuis Gent, Ghent, Belgium
- Emilios K. Dimitriadis, PhD, Biomedical Engineering & Physical Science Shared Resource Program, NIBIB, Bethesda, MD
- Antonella Forlino, PhD, Università degli Studi di Pavia, Pavia, Italy
- Ken Kozloff, PhD, University of Michigan, Ann Arbor, MI
- Jennifer A. Lippincott-Schwartz, PhD, Advanced Microscope Facility, NICHD, Bethesda, MD
- Joan C. Marini, MD, PhD, Section on Heritable Disorders of Bone and Extracellular Matrix, NICHD, Bethesda, MD
- Charlotte L. Phillips, PhD, University of Missouri, Columbia, MO
- Pamela G. Robey, PhD, Craniofacial and Skeletal Diseases Branch, NIDCR, Bethesda, MD
- Antonio Rossi, PhD, Università degli Studi di Pavia, Pavia, Italy
- Constantine A. Stratakis, MD, D(med)Sci, Section on Endocrinology and Genetics, NICHD, Bethesda, MD
Contact
For more information, email leikins@mail.nih.gov or visit http://physbiochem.nichd.nih.gov.