Regulation of Retrograde Axonal Transport in Developing and Mature Neural Circuits

- Catherine Drerup, PhD, Head, Unit on Neuronal Cell Biology
- Amrita Mandal, PhD, Visiting Fellow
- Dane Kawano, BSc, Postbaccalaureate Fellow
- Wesley Schnapp, BSc, Postbaccalaureate Fellow
- Katherine Pinter, BSc, Technician
The overarching theme of our lab is to determine the mechanistic regulation of retrograde transport in neurons and the functional ramifications of disrupting this process. Intracellular transport is critical in all cells of the body to form and maintain functional organ systems. Neurons are particularly reliant on this process, however, owing to their large size and high metabolic demand. Axons, the primary projection from the neuronal cell body to distant targets, can extend great distances from the cell body, where most proteins are produced. For example, axons that make up the sciatic nerve in humans can extend approximately a meter from the cell body to innervate the distal appendages. This makes the relative axon length to cell body diameter ratio approximately 100,000:1. In order to form this large and complicated structure, proteins and organelles must be continually shipped from the cell body through axons. This intracellular movement, termed axonal transport, utilizes molecular motors that move on cytoskeletal tracks to deliver cargo to the correct sub-cellular location. While anterograde (axon-terminal directed) transport utilizes a superfamily of motor proteins, retrograde (cell-body directed) transport utilizes a single motor protein complex called cytoplasmic dynein (Figure 1). We use genetics, biochemistry, and in vivo imaging in zebrafish to identify cargo-specific regulators of retrograde axonal transport. Subsequently, we can use these tools to determine how disruptions in cargo retrograde movement impact the stability and function of the neural circuit in vivo. Perhaps not surprisingly, disruptions in axonal transport have been correlated with numerous developmental and degenerative diseases of the nervous system, including Charcot-Marie-Tooth disease, Alzheimer's disease, Parkinson's disease, Huntington's disease, amyotrophic lateral sclerosis, and hereditary spastic paraplegia, to name a few. However, whether axonal transport abnormalities are causal in disease progression is still largely unknown. Our combination of approaches, which rely on the unique advantages of the zebrafish system, will permit us to answer long-standing questions regarding the relationship between axonal transport regulation and disease pathology.
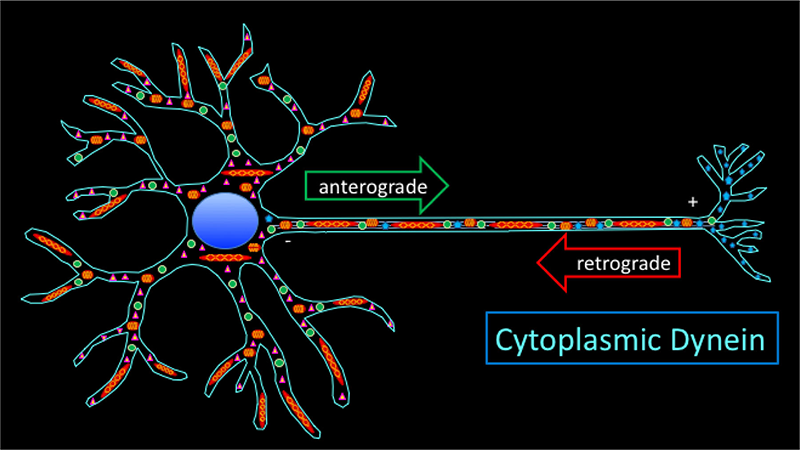
Click image to enlarge.
Figure 1. Axonal transport and the neuron
Axonal transport is essential to move cargos, including proteins and organelles, between the neuronal cell body and axon terminals. While members of the kinesin superfamily of molecular motors move cargoes towards microtubule plus ends in axon terminals, a single motor protein complex, cytoplasmic dynein, is the primary motor required for retrograde cargo transport in axons. As the complicated subcellular architecture of neurons is required for their function, regulated movement of intracellular components to the proper location is essential for the formation and maintenance of functional neural circuits.
Mitochondrial transport, health, and function in axons
Mitochondrial transport is necessary to properly position this organelle in axons. Correct mitochondrial localization in axons is critical for energy production near sites of high metabolic demand, for calcium homeostasis to regulate neuronal activity, and to regulate axonal branching in certain contexts. Various model systems have revealed several mediators of mitochondrial movement. Anterograde mitochondrial transport requires the Kinesin-1 molecular motor in association with several other proteins, including Miro and Milton. Interestingly, loss of Miro or Milton, the two best characterized adaptors for mitochondrial transport, eliminates all mitochondrial movement. Therefore, how this organelle is selectively transported in the retrograde direction is still unclear.
In a forward genetic screen, we identified a novel zebrafish mutant strain with selective loss of retrograde mitochondrial transport. The causative mutation in this line causes loss of a protein known to associate with the dynein motor Actr10 (Figure 2). In actr10 mutants, anterograde mitochondrial transport is intact but retrograde mitochondrial transport frequency is dramatically reduced, leading to accumulation of mitochondria in actr10 mutant axon terminals. Using mitochondrial fractionation, we demonstrated that the loss of retrograde transport frequency is attributable to loss of mitochondria-dynein interaction in the absence of Actr10. We are currently using the actr10 mutant as a tool to determine the impact of mitochondrial retrograde transport disruption on mitochondrial health and function. Additionally, we are pursuing the impact of mitochondrial accumulation on the health and function of the axon. Together, the work will define the molecular mechanisms of retrograde mitochondrial transport and provide insights into how loss of this specific cellular activity impacts the organelle, the cell, and the neural circuit in vivo.

Click image to enlarge.
Figure 2. The dynein-dynactin complex
The core dynein motor is composed of heavy chains (HCs) with ATPase domains and a microtubule interaction domain. In addition, light chains (LCs), light intermediate chains (LICs), and intermediate chains (ICs) make up this complex. Dynein is bound to the accessory complex dynactin through interaction of p150 with the IC tails. Actr10, a component of the dynactin pointed end complex, is lost in the mutant line with disrupted mitochondrial retrograde transport.
Screening for novel regulators of cargo-specific retrograde axonal transport
Several pieces of evidence substantiate the importance of retrograde axonal transport for axon health and function. First, mutations in dynein and dynein-associated proteins are correlated with neurological disease. Second, retrograde transport of signaling endosomes is essential for the extension and maintenance of long axons. Third, abnormal localization of various cargoes, including mitochondria, correlates with neuronal disease. Despite the importance of the process, little is known about how various cargoes attach to and then are transported by the retrograde motor protein complex. We are using forward and reverse genetics in zebrafish to identify mediators of dynein-specific retrograde transport in axons.
Using a double transgenic line in a three-generation, forward genetic screen, we are identifying recessive mutant strains with axon abnormalities characteristic of disruptions in retrograde axonal transport. Our previous work and that of others showed that these phenotypes include axon terminal swellings such as those observed in actr10 mutants (Figure 3). After identifying the strain, we use RNA–sequencing approaches to identify the causal mutation. To date, we have identified three lines, which all have mutations in dynein-associated proteins. At present, we are using immuno-labeling approaches and in vivo imaging techniques we previously developed to determine whether these strains have deficits in the retrograde transport of specific cargoes. With these mutant strains we can first identify the proteins involved in the retrograde transport of particular cargoes and then use the strains as tools to determine how the specific disruptions affect the function of the axon.
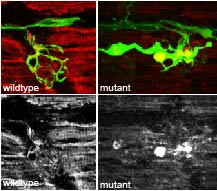
Click image to enlarge.
Figure 3. Cargo accumulation resulting from disrupted retrograde transport is associated with axon terminal swellings.
Axon terminal in a wild-type zebrafish larvae at 4 days post-fertilization (dpf; left). Axons are marked by the neurod:egfp transgene (green). Cytochrome C staining marks mitochondria in the axon terminal (red on top, white on bottom). An actr10 mutant at 4 dpf has axon terminal swellings with mitochondrial accumulation resulting from disrupted retrograde mitochondrial transport.
Publications
- Drerup CM, Herbert AL, Monk KR, Nechiporuk A. Regulation of mitochondria-dynactin interaction and mitochondrial retrograde transport in axons. eLife 2017 10.7554/eLife.22234.
- Drerup CM, Nechiporuk A. In vivo analysis of axonal transport in zebrafish. Methods Cell Biol 2016 131:311-329.
- Herbert AL, Fu MM, Drerup CM, Gray RS, Harty BL, Ackerman SD, O’Reilly-Pol T, Johnson SL, Nechiporuk AV, Barres BA, Monk KR. Dynein/dynactin is necessary for anterograde transport of Mbp mRNA in oligodendrocytes and for myelination in vivo. Proc Natl Acad Sci USA 2017 114:E9153-E9162.
Contact
For more information, email katie.drerup@nih.gov or visit http://dreruplab.nichd.nih.gov.