Building the Zebrafish Lateral Line System
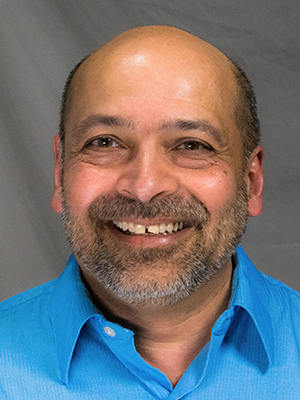
- Ajay Chitnis, MBBS, PhD, Head, Section on Neural Developmental Dynamics
- Damian E. Dalle Nogare, PhD, Staff Scientist
- Gregory Palardy, BS, Research Technician
- Chongmin Wang, MS, Research Technician
- Harsha Mahabaleshwar, PhD, Postdoctoral Fellow
- Uma Neelathi, PhD, Postdoctoral Fellow
- Caitlin Fox, BS, Graduate Student
- Alexandria Bikker, BS, Postbaccalaureate Intramural Research Training Award Fellow
- Marci Rosenberg, BS, Postbaccalaureate Intramural Research Training Award Fellow
Our current studies examine how the posterior lateral line system is built in the zebrafish nervous system. Our goal is to define the genetic regulatory network that coordinates cell fate and morphogenesis in the lateral line system and to build computational models that help us understand how this relatively simple and extremely accessible sensory system in zebrafish builds itself.
The lateral line is a mechanosensory system that detects water flow and consists of sensory organs called neuromasts, which are distributed in a stereotypic pattern over the surface of the zebrafish. Each neuromast has sensory hair cells at its center, which are surrounded by support cells that serve as progenitors for the production of more hair cells during the growth and regeneration of neuromasts. The development of this superficial sensory system in zebrafish can be easily observed in live embryos carrying transgenic lines expressing fluorescent proteins in specific subsets of cells of the lateral line system. In addition, a range of genetic and cellular manipulations can be used to investigate gene function.
The function of sensory hair cells in fish neuromasts is remarkably similar to that of hair cells in the vertebrate ear. Furthermore, the gene-regulatory network that determines specification of neuromast hair cells is very similar to the one specifying hair-cell fate in the human ear. Like the hair cells in our ears, neuromast hair cells can be damaged by exposure to drugs such as aminoglycosides, to copper ions, and to loud sounds. However, unlike our ears, in which the loss of hair cells can be permanent, zebrafish neuromast hair cells have a remarkable ability to regenerate. Hence, the lateral line system serves as an excellent model system for understanding development and for developing strategies to engineer regeneration of sensory hair cells.
The posterior lateral line system is initially established by the posterior lateral line (pLL) primordium, a cluster of about a 140 cells that migrate from the ear to the tip of the tail, periodically depositing neuromasts. Recent studies showed that the mechanisms that determine and guide collective migration and deposition of cells from the pLL primordium are remarkably similar to those that determine the collective migration of metastatic cancer cells. Hence, the lateral line system has also recently emerged as an excellent system for studying the biology of metastatic cancer cells.
Our expectation is that understanding the genetic regulatory network that coordinates cell fate in and morphogenesis of the zebrafish lateral line system will ultimately have a profound impact on translational studies that address a wide range of issues, including the development and regeneration of sensory systems and therapies directed at limiting the spread of cancer through metastasis.
Self-organization of the zebrafish lateral line primordium
Interactions between the Wnt (a signaling protein), FGF (fibroblast growth factor), Notch (a transmembrane receptor), BMP (bone morphogenetic protein), and chemokine signaling pathways in the pLL primordium provide a framework for understanding how cell fate and morphogenesis are coordinated in this group of about a hundred cells, as they collectively migrate under the skin from the ear to the tip of the tail. We combined an experimental approach with computational modeling to define the mechanisms that determine both the periodic formation of neuromasts and their deposition along with inter-neuromast cells, as the pLL primordium migrates along a path defined by chemokine expression.
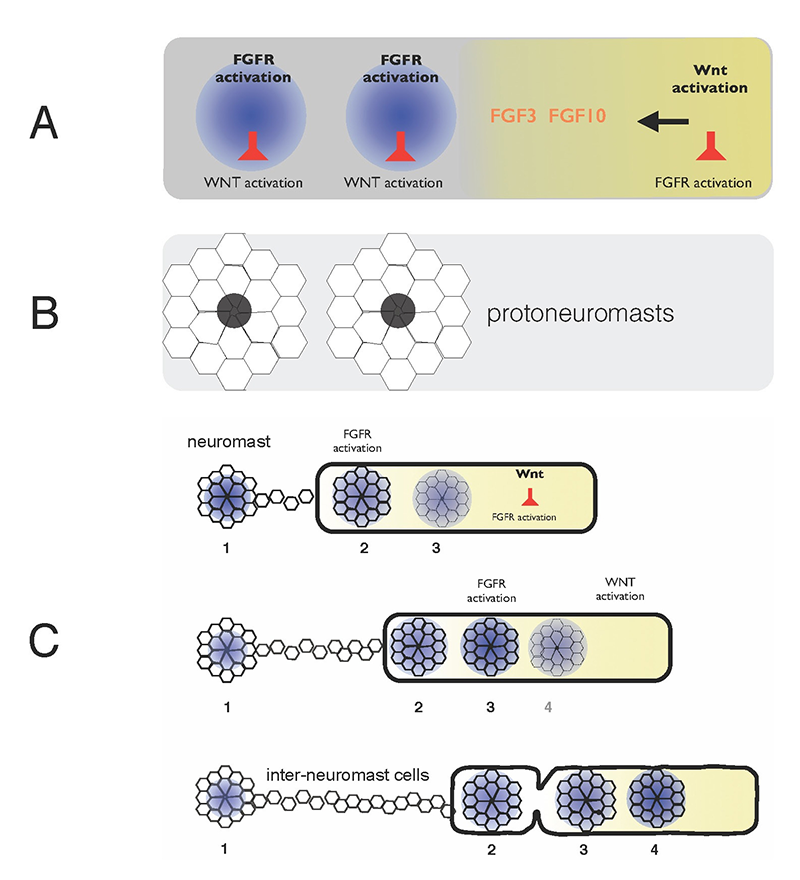
Click image to enlarge.
Figure 1. Self-organization of zebrafish lateral line primordium
A. FGFs secreted in response to Wnt activity in a leading zone (yellow) activate FGF receptors (FGFRs) in the trailing zone (blue). Wnt activity inhibits FGFR activation, and FGFR activation induces expression of a secreted factor that inhibits Wnt activity.
B. Activation of FGFRs coordinates formation of protoneuromasts, in which cells reorganize to form epithelial rosettes, and a central cell (black) is specified as a sensory hair-cell precursor.
C. Once about two neuromasts form, the primordium starts migrating. The Wnt zone (yellow) progressively shrinks as new protoneuromasts form ever closer to the leading end. As the Wnt system shrinks, so does the primordium, and neuromasts and interneuromast cells are shed from the trailing end.
Wnt proteins initially activate Wnt-beta catenin in a broad leading zone of the primordium. Cells with Wnt activity respond by becoming a source of FGF ligands. At the same time, they express factors that prevent a response to these FGFs. As leading cells with relatively high levels of Wnt activity are prevented from responding to the FGFs, an FGF–responsive center is initially established at the trailing end of the pLL primordium, where Wnt activity is weakest. The activation of FGF receptors in these trailing cells coordinates the formation of nascent neuromasts, where cells reorganize to form epithelial rosettes, and a central cell is specified as a sensory hair-cell progenitor. However, the FGF–responsive center also becomes the source of a secreted Wnt antagonist, which progressively restricts the domain of active Wnt activity to the smaller leading zone. The shrinking Wnt system allows the formation of another FGF signaling–dependent ‘protoneuromast’ in its wake. In this manner, as the Wnt system shrinks, new protoneuromasts are formed, progressively closer to its leading end. After about the first two protoneuromasts form, the primordium starts migrating toward the tail.
As the pLL primordium migrates, cells are deposited from its trailing end, and the pLL primordium progressively shrinks. Cells that were incorporated into protoneuromasts are deposited as neuromasts, while cells that were not effectively incorporated into epithelial rosettes are deposited as interneuromast cells. Interestingly, shrinkage of the pLL primordium correlates with shrinking of the Wnt system, and the length of the Wnt active zone is always roughly 60% of the length of the primordium. Knowing the initial size of the Wnt system, and the rate at which it shrinks, provides a way to predict the permitted length of the primordium at any point during the course of migration. Trailing cells in a position that exceeds the permitted length of the primordium slow down, stop migrating, and are shed from the trailing end. The rate at which cells leave the migrating primordium depends on the rate at which the Wnt system shrinks and on the cell proliferation rate, as addition of cells adds to the length of the primordium, and hence to the possibility that trailing cells will fall outside the permitted length of the migrating primordium. We used this framework to build a computational model that effectively predicts how far the pLL primordium will migrate, how many neuromasts it will deposit and the length, on average, of the spacing between deposited neuromasts. A comparison of data from both wild-type embryos and lef1 mutant embryos, in which the proliferation is half that of wild-type embryos and migration speed progressively decreases, showed that the computational model uses a few simple parameters to make fairly accurate predictions about the pattern of neuromast deposition by the migrating primordium.
While the framework described above provides a broad description of the early self-organization of the PLLp system, many questions remain. In recent years, we have focused on the following questions: (1) the precise lineage of cells in the migrating primordium and how cells in different locations contribute to the sequential formation of neuromasts and to specific fates within deposited neuromasts; (2) the mechanisms that determine the dynamics of Wnt-FGF signaling in the pLL primordium, including those that determine its initial polarization, progressive shrinking of the leading Wnt system, and sequential formation of trailing FGF signaling centers in its wake; (3) the signaling mechanisms that determine collective migration of the pLL primordium and how these signaling systems are linked to the mechanics of migration; (4) what the behavior of individual cells within the primordium reveals about the mechanisms of collective migration and cell-cell communication; (5) whether what has been learned from these studies will allow us to build computational models and a theoretical framework that helps reconstruct how specific interactions between cells and their environment result in the coordination of morphogenesis, cell fate, and migration of the PLLp and, importantly, whether failure of some of our models to recapitulate specific phenomena helps identify gaps in our understanding and define questions for future examination.
Characterizing cell migration, proliferation, lineage, and fate specification in the migrating PLLp at single-cell resolution
Many basic aspects of the biology of the PLLp, including proliferation patterns, lineage relationships, and the dynamics of neuromast deposition remain incompletely characterized. To unambiguously resolve such questions, we conducted time-lapse imaging of the PLLp, first at low resolution for some embryos, and then again for three independent embryos at a resolution that allowed us to track and catalog every single cell in the PLLp from early in migration until termination (approximately 24 hours). From this dataset, we constructed digital models of the PLLp. We could query these models to define the lineages of specific cells or subsets of cells, such as those that form specific neuromasts or that adopt specific fates within the neuromasts. Previous studies had suggested that the leading zone with Wnt activity maintains a proliferative population of progenitor cells that divide to produce a subset of daughter cells that move to a trailing zone, where, under the influence of FGF signaling, they differentiate to sequentially form neuromasts. However, our analysis revealed that proliferation in the PLLp is largely unpatterned and not especially high in the leading zone. Furthermore, protoneuromasts are not formed in the trailing zone from a self-renewing population of progenitors in a leading zone. Instead, they are formed by the local proliferation of cells along the length of the primordium in response to FGF signaling, as the Wnt signaling system, which locally inhibits protoneuromast formation, is progressively restricted to a smaller leading zone of the PLLp. The fate of deposited cells, within neuromasts, as central sensory hair cell progenitors, surrounding support cells, or as interneuromast cells between deposited neuromasts, is not determined by any obvious stereotyped lineages. Instead, their fate is determined, somewhat stochastically, as a function of a cell’s distance from the center of a maturing protoneuromast. This observation is consistent with the notion that a cell’s fate is determined in the PLLp by its level of exposure to active FGF signaling; the central cell in the protoneuromast, most likely specified earlier as a sensory hair cell progenitor by its exposure to the highest level of FGF signaling and via lateral inhibition mediated by Notch, becomes a source of FGF10. We suggest that proximity to this new central source of FGFs subsequently determines the level of FGF signaling and the eventual fate of surrounding cells in the maturing protoneuromast.
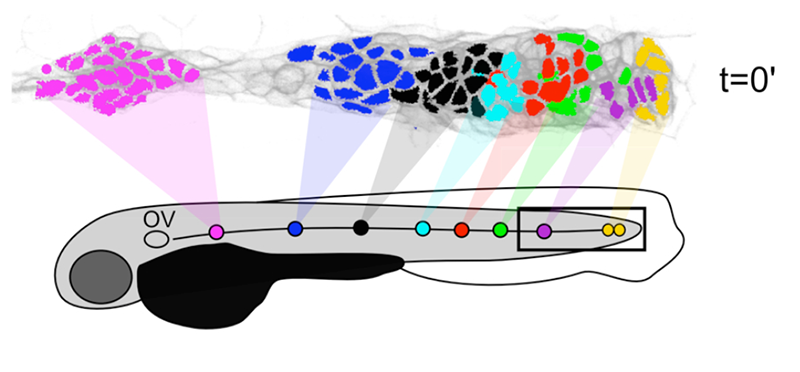
Click image to enlarge.
Figure 2. Neuromasts are formed by the local proliferation of cells along the length of the primordium.
Filled circles represent sequentially deposited neuromasts. Corresponding colors of cells in the PLLp at time t=0′ show which populations underwent local expansion to give rise to each of these neuromasts.
Publications
- Dalle Nogare D, Chitnis AB. A framework for understanding morphogenesis and migration of the zebrafish posterior Lateral Line primordium. Mech Dev 2017 148:69-78.
- Nogare DD, Nikaido M, Somers K, Head J, Piotrowski T, Chitnis AB. In toto imaging of the migrating Zebrafish lateral line primordium at single cell resolution. Dev Biol 2017 422:14-23.
- Knutsdottir H, Zmurchok C, Bhaskar D, Palsson E, Dalle Nogare D, Chitnis AB, Edelstein-Keshet L. Polarization and migration in the zebrafish posterior lateral line system. PLoS Comput Biol 2017 13:e1005451.
Collaborators
- Hari Shroff, PhD, Laboratory of Molecular Imaging and Nanomedicine, NIBIB, Bethesda, MD
Contact
For more information, email chitnisa@mail.nih.gov or visit https://irp.nih.gov/pi/ajay-chitnis.