The Regulation or Disturbance of Protein/Lipid Interactions in Influenza, Malaria, Diabetes, Muscular Dystrophy, Brain Trauma, and Obesity
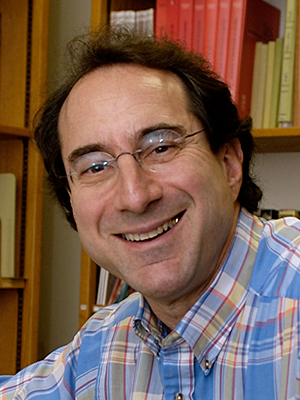
- Joshua Zimmerberg, MD, PhD, Head, Section on Integrative Biophysics
- Paul S. Blank, PhD, Staff Scientist
- Svetlana Glushakova, MD, PhD, Staff Scientist
- Petr Chlanda, PhD, Visiting Fellow
- Matthias Garten, PhD, Visiting Fellow
- Sourav Haldar, PhD, Visiting Fellow
- Brad Busse, PhD, Postdoctoral Intramural Research Training Award Fellow
- Chad McCormick, PhD, Postdoctoral Intramural Research Training Award Fellow
- Ludmila Bezrukov, MS, Chemist
- Hang Waters, MS, Biologist
- Elena Mekhedov, MA, Contractor
- Tatyana I. Tenkova-Heuser, PhD, Contractor
- Glen Humphrey, PhD, Guest Researcher
- John E. Heuser, MD, Senior Biophysicist
- Jennifer Petersen, PhD, Electron Microscopist
Fusion and fission, the instances when organelles gain or lose their identities, are the essence of complex membrane dynamics in living cells and are key elements of synapses and other dynamic cellular trafficking networks. Without fusion and fission, enveloped viruses and parasites could not enter cells, replicate, or exit cells, nor would inflammatory cells respond and kill such invaders or deal with sick cells. Our earliest work concentrated on model membrane systems, the physical properties and theoretical pathways required for membrane fusion to occur, and the discovery that tension spreads headgroups for hemifusion, then pulls open fusion pores to allow coalescence of adherent bilayers. However, while able to focus on basic membrane biophysical properties and help develop a theoretical framework to understanding membrane interactions, model systems were a simplification that ignored the important roles of proteins. Including the role of proteins in these fundamental biophysical processes was both fruitful and informative, culminating in what we believed to be a canonical framework for understanding both fusion and fission. We introduced a simple paradigm: proteins act as catalysts (bilayer topoisomerases) lowering the huge energy barriers to membrane remodeling steps. A few amino acids of a specialized protein domain can reversibly enter the hydrophobic membrane matrix or cover the headgroups as inclusions or scaffolds, respectively, and thus transiently alter the thermodynamics of the system by specific protein-lipid interactions. By combining quantitative light microscopy with electrophysiology, and reconstitution of fusion and fission in lipid bilayer membranes, we constructed hypotheses with predicted fusion intermediates whose dimensions were deduced by continuum theory and fits to experiments. The predicted sizes were detectable by cryo-electron microscopy, so we labored to achieve the highest-resolution electron microscopy of hydrated membrane fusion events in order to understand how proteins catalyze the new configurations of lipids that ultimately mediate these processes. By successfully installing a new technology at NIH, the Volta Phase Plate, we were able to visualize the predicted hemifusion diaphragm mediated by the hemagglutinin (HA) of influenza virus (IFV), and the measurements of its dimensions fit the predictions of continuum theory. However, another result was unexpected: HA catalyzed the breakage of membranes, leading to free membrane edges—often in great profusion.
To understand why this was unexpected, we must consider the physical forces that act on lipids in solution. Membranes avoid edges. The lipid bilayer is self-assembling because its free energy of cohesion (which derives in part from enthalpic attractive forces between hydrocarbon chains and in part from the entropic hydrophobic effect that minimizes interfacial area) automatically ensures stability of the lipid bilayer. Formally, the edge of an otherwise lamellar membrane has a large linear tension, i.e., should be a high-energy region that the membrane seeks to minimize. Nevertheless, we observe that ‘free edges’ do indeed outnumber hemifusion diaphragms for certain lipid compositions of target membranes. Such edges only occur in close vicinity to activated HA molecules, indicating that edges are triggered to form by the same event that triggers full fusion: namely, the amphipathic helix of HA being ejected from HA and binding to the target bilayer. We can only presume for the moment that the HA fusion peptide somehow stabilizes the observed membrane edges, i.e., drastically lowers bilayer line-tension. This observation and resultant hypothesis forms the basis of our future work.
Membrane fusion, fission, and the role of cholesterol in the assembly of influenza virus
There are two threads that run through all of the Section’s work: the use of quantitative measurements of dynamic systems to test hypotheses deduced from biophysical reasoning. Our impact goes beyond our single experiment when we develop new technology that paves the road to future work. In the past, capacitance measurements opened up the field of the fusion pore to experimentation, as did simultaneous imaging with electro-physiology, and laser excitation of photo-activatable fluorophores opened up single-molecule imaging to living cells. More recently, the implementation of electrical and fluorescent measurements of cylindrical lipid nanotubes allowed us to test hypotheses regarding the interaction of dynamin and membrane curvature–scaffolding proteins with membranes. We found that the GTPase dynamin acts as a scaffold with many small amino acid insertions into the outer monolayer of a lipidic structure called the fission pore, to form a hemi-fission intermediate. The hemi-fusion intermediate was induced by influenza virus HA incorporating in virosomes and interacting with targeted lipid vesicles at low pH. Ultra-thin films were plunge-frozen and visualized by Volta phase plate cryo-electron tomography (VPP-cET). We identified two distinctly different hemifusion structures: a hemifusion diaphragm and a highly unexpected novel structure termed a ‘lipidic junction’ (Reference 1). The edges of liposomes’ lipidic junctions were ruptured and stabilized by HA. The high frequency of lipidic junctions exclude their artefactual origin. Both rupture frequency and hemifusion diaphragm diameter decreased when the liposome cholesterol level matched physiological concentrations.
Another unexpected ultrastructural observation came when we deacylated HA, leading to our new hypothesis that HA acylation contributes to membrane curvature in influenza virus A assembly and HA–mediated membrane fusion. Indeed, de-acylation of the cytoplasmic tail of HA interrupted interactions between HA and matrix (M1) and affected viral envelope curvature, without influencing viral-like particles’ (VLP) shape, lipid composition, or HA lateral spacing. Observed VLP size distribution data were supported by molecular dynamic simulations data based on the spontaneous curvature of palmitate. HA acylation also controls curvature-dependent fusion-pore widening, an important step in membrane fusion. In addition, HA acylation regulates the binding of M1 matrix protein on the budding virus during assembly. Thus, HA acylation contributes to the ability of HA to control curvature to bend membranes and control curvature-dependent fusion-pore widening, emphasizing the importance of protein-lipid interactions in membrane remodeling (Reference 2). For wild-type HA, the interaction of palmitate augments the natural electrostatics of M1/surface binding to negatively charged envelope. The layering and subsequent uncoating of influenza M1 at low pH provides enough tension to rupture membranes. In neutral media, adsorption of M1 protein to a lipid bilayer is strong, electrostatic, and reversible. Acidification alters M1 charged groups, driving a conformational change and partially desorbing M1 from the membrane because of increased repulsion between remaining M1. Lipid vesicles coated with M1 ruptured at low pH owing to this repulsive force. These studies indicate how protein motifs and their interactions with lipids can create the forces required for membrane transformations driven by fission, fusion, and lipidic edge formation.

Click image to enlarge.
HA acylation affects curvature of the released particles.
(A) Representative slices of tomograms showing MAYNA (no M1), HANA (no M1), and HANAM1M2 spheroidal particles. Respective average radii, standard deviations, and numbers of measurements are shown below the images.
(B) Peak normalized probability density functions (PDF) for the three species, HA with and without M1 and MAY without M1, calculated using the truncated Gaussian parameters identified from the global fit of the cumulative distributions of VLP radii. HA and MAY differ in their most probable size (peak of the PDF), and the distribution width is significantly greater in the absence of M1.
(C) Spontaneous curvature of palmitic acid and palmitoylation on short alpha-helical peptide sequences computed from simulation. Extrapolation of the data to the 100% area fraction of palmitic acid yields an estimate of the spontaneous curvature of palmitic acid. Shown for reference (red point on the right curvature axis) is the same value estimated from the change in average size of the VLPs with HA compared to that with MAY.
Abbreviations: MAYNA, mutated neuraminidase (NA) in which the mutant amino acids are M, A, and Y; HANA, viral particle made with wild-type HA and NA; M1 and M2, matrix proteins; MAY, mutant HA.
To understand the origin of the negative charged lipids within the viral envelope, a fusion protein/lipid interactome project aimed to assess the intimate relationship of viral fusion protein with the biological membrane at a nm spatial scale. Cholesterol-sphingolipids distribution was linked to influenza virus budding. Secondary ion mass spectrometry (SIMS) was employed to image, with about 100 nm lateral resolution, the distributions of isotope-labeled cholesterol and labeled viral protein in the plasma membrane during virus budding (ongoing work). The data show that, in MDCK cells (mammalian cell-culture cells), influenza virus does not bud from cholesterol-enriched membrane domains. However, sphingolipid domains need not be enriched with cholesterol. SIMS was used to map the distributions of isotope-labeled cholesterol and sphingolipids in the plasma membranes of fibroblast cells; HA clusters were neither enriched with cholesterol nor co-localized with sphingolipid domains. HA clustering and localization in the plasma membrane is not controlled by cohesive interactions between HA and liquid-ordered domains enriched with cholesterol and sphingolipids, or from specific binding interactions between hemagglutinin, cholesterol, and/or the majority of sphingolipid plasma membrane species.
Membranes during egress of the causative agent of malaria
Malaria continues to devastate children worldwide, with over 300,000 deaths from malaria in children under five in 2015 alone. With no vaccine available and drug resistance climbing, we are focusing on the unique membrane biology of the parasites that cause malaria, to find new targets for therapy. In our work, methods have been compiled allowing a comprehensive quantitative evaluation of parasite replication in erythrocytes. By developing, publishing, and promulgating new methods to study the biology of the malaria parasite, our work has impacted the field by transforming qualitative imaging to quantitative measures, providing the first recordings of P. falciparum egress and invasion of erythrocytes, describing new phenomena such as shape transformation of infected cells signaling the egress initiation and membrane transformation upon egress. We developed several non-interventional methods that allow fine staging of cell phenotype and quantification of the parasite replication cycle as it naturally progresses from parasite invasion of erythrocytes to parasite egress from the host cells. As a result, we were able, for the first time, to assess an intimate relationship between egress and invasion steps in parasites defective in an egress/invasion protease cascade. In addition, we corrected several mistaken conclusions about parasite biology and drug effects, by avoiding artifacts caused by the mechanical and osmotic fragility of live, infected erythrocytes (iRBC) and their exceptional sensitivity to illumination.
The methods we developed include: (1) quantitative assay for parasite intra-erythrocytic multiplication factor (IMF), which is the first assay to quantify the number of progeny per individual cell and thus permits precise assessment of the role of parasite and host on parasite multiplication; (2) quantitative assay of the length of parasite cycle, which permits assessment of the effects of any intervention into parasite physiology on cycle progression and host-cell biophysical characteristics, such as volume, hemoglobin content, cell hydration, ion homeostasis (the assay also provides cell phenotyping and timing of any physiological block to cycle progression); (3) quantitative parasite egress assay for short-duration treatments of iRBC allows testing of ionophores or other fast-acting drugs or conditions on parasite egress, extending the range of interventions into parasite physiology that now can be quantified; (4) assessment of photo-damage to iRBC by inhibition of parasite egress from erythrocyte upon controlled cell illumination (intensities and wavelength) to extend the parameters for microscopic work without damage; (5) a new method to release intact parasitophorous vacuoles (PV) from live iRBC for electrophysiological studies on the PV membrane using a patch clamp technique, a new method that exploits the K+ permeability of infected erythrocytes, which swells the PV till it ejects from the iRBC, with vacuolar membranes intact (i.e., vacuoles preserve their vacuolar content) and easily patched by glass pipets; (6) quantitative parasite invasion assay, which allows naturally egressed parasites to invade neighboring normal erythrocytes (Reference 3), an invasion method that is independent of the parasite egress rate and is able to test pulse exposure to drugs (15–30 min), thus excluding the effect of drugs on parasite development.
Using these methods, new targets continue to be discovered in Plasmodium falciparum, the most clinically devastating species. The most promising to date is a pair of ‘druggable’ mediators of parasite egress and invasion, namely, aspartic proteases Plasmepsins IX and X. We determined that the aspartic protease PMIX (acting from within the ‘rhoptry’, apical secretory organelles of Plasmodia) is essential for erythrocyte invasion (Reference 3). In contrast, PMX, by controlling maturation of the subtilisin-like serine protease SUB1 in exoneme secretory vesicles, is essential for both egress and invasion. A lead compound, C-117, is currently under intense evaluation by pharmaceutical companies, because it works in the high nanomolar range and is well tolerated orally by mice (Reference 3). In another study, we showed a new potential route for drug delivery thought to be impossible. Despite its membrane impermeability, the natural glycosaminoglycan heparin inhibited malaria parasite egress, trapping merozoites within infected erythrocytes (Reference 4). Heparin does not bind to the erythrocyte surface, rather it enters iRBC at the last minute of the parasite cycle through parasite-induced pores that we discovered in iRBC. This short encounter was sufficient to significantly inhibit parasite egress and dispersion. Heparin blocks egress by interacting with both the surface of merozoites and the inner aspect of erythrocyte membranes, preventing the rupture of infected erythrocytes but not of parasitophorous vacuoles, and independently interfering with merozoite disaggregation. Given that this action of heparin offers a plausible explanation of how neutralizing antibodies can block egress, we intend to exploit membrane perforation as a new physiological strategy to target therapeutics intracellularly (Reference 4). While we continue to search for the molecular identity of the pores before merozoite egress, we learned that a perforin-like protein, PPLP2, permeabilizes the iRBC membrane during gametocytes egress. Like merozoites, egress of malaria gametocytes from iRBC requires the concerted rupture of vacuolar and erythrocyte membranes. We showed that PPLP2 is essential for normal egress of gametocytes from iRBC but that it is dispensable for parasite asexual cycle. PPLP2– gametocytes were trapped within erythrocytes owing to failure to permeabilize the erythrocyte membrane despite a normal disruption of the vacuole membrane. As a consequence, transmission of PPLP2– parasites to the Anopheles vector was reduced. Thus, the hemolytic activity of PPLP2 is essential for gametocyte egress and transmission to mosquitoes.
One clinical issue for patients with malaria relates to its pathophysiology in hematological disorders. By using our IMF assay (above) to quantify the number of merozoites released from an individual schizont, and infecting blood, lab or clinical isolates, from patients with various thalassemias or sickle disease with P. falciparum, we determined that IMF strongly correlates with hemoglobin concentration of the host cells. We undertook mathematical modeling of malaria progression to learn that a lower IMF limits parasite density and anemia severity over the first two weeks of parasite replication. Thus, P. falciparum IMF is a parasite heritable virulence trait that correlates with erythrocyte indices and is reduced in thalassemia-trait erythrocytes.
By using the above photo-damage assay, we showed that low fluence red light is the safest to study parasite physiology with continuous or time lapse microscopy. Photo-sensitizing fluorescent dyes should be used with extreme caution in the study of cell physiology of malaria parasites. We are developing a comprehensive method to assess the malaria parasite replication cycle, using live cell microscopy that takes into consideration the photosensitivity of iRBC.
Muscle membrane modification to treat muscular dystrophy
A major theme under development is that membrane transformations and dynamics, including the newly identified membrane “edge”, are strongly influenced by lipid composition. To extend our research to physiology and pathophysiology, we explored the possibility that lipid composition can be altered with diet. We designed a dietary intervention trial in the dysferlin-deficient A/J mouse (a model of muscular dystrophy), which develops a mild myopathy after 6 months of age, to test whether a diet rich in alpha-linoleic acid alters lipid content by an iso-caloric substitution of flaxseed oil for soybean oil in a standard defined diet. After five months, the test (flax) group had greater weight and muscle gain than the soy group, with no evidence for detrimental side effects. Lipidomics analysis of muscle tissue from the two diet groups demonstrated that levels of arachidonic acid (AA)–containing phospholipids were four-fold lower, and levels of alpha-linoleic acid–containing phospholipids were four-fold higher, in the flax-diet group. The results indicate that membrane lipid composition can be modified through changes in diet. While evidence exists for a beneficial role for dietary omega-3 fatty acids, less is known about other fatty acids or analogs with potential therapeutic benefit when incorporated into lipids. One such fatty acid is phytanic acid, which can be incorporated into membrane lipids. However, the ability of phytanic acid to modify biological membranes through a diet that would incorporate such stabilizing lipids into membranes was unknown. In addition, methodologies to quantify the incorporation of phytanic acid were not developed. We therefore initiated both a dietary study to evaluate phytanic acid incorporation into phospholipids and a new technique to measure phytanic acid species: ion mobility mass spectrometry. Dysferlin-deficient A/J mice were maintained on a defined diet supplemented with 2% phytol (a metabolite of which is phytanic acid) for three weeks. The muscle tissue was then analyzed using ion mobility mass spectrometry. Lipid species were differentially detected in the phytol diet muscle (m/z 790.7 and 862.7) and tentatively identified as phosphatidylcholine (PC) 20:0-16:0 and PC 20:0-22:6. To confirm the presence of phytanic acid, the ion mobility of these species was compared with standards, and determined to be a PC having one phytanic acid chain. We estimate that these species represent 10% of PC, validating a dietary strategy in altering muscle membranes. These ‘proof-of-principle’ studies in dietary changes and mass spectrometry measurements of membrane lipids advance our ability to study the effects of specific lipids on membrane stability and to test lipids as therapies in muscular dystrophy.
Technological advances in this arena were focused on imaging muscle and protein relations within muscle. (1) Continuing our long-standing contributions to super-resolution microscopy, we found that adaptive optics improves multiphoton super-resolution imaging. Thus, using a reference beam to determine optical aberrations and a deformable mirror to correct them, signal intensity was increased up to 40-fold with a lateral resolution down to 176 nm at depths up to 0.25 mm in embryos and other tissues (Reference 5). (2) Fluorescent proteins are in the static regime when excited; in this regime, estimating separations between Förster resonance energy transfer (FRET) pairs is problematic. We exploited the experimentally measured average FRET efficiency to estimate separations between fluorescent proteins.
Additional Funding
- 2017 Deputy Director for Intramural Research (DDIR) Innovator’s Award
- NIH Intramural-to-Russia (I-to-R) Program Award
- Office of AIDS Research (OAR) Award
Publications
- Chlanda P, Mekhedov E, Waters H, Schwartz CL, Fischer ER, Ryham RJ, Cohen FS, Blank PS, Zimmerberg J. The hemifusion structure induced by influenza virus haemagglutinin is determined by physical properties of the target membranes. Nat Microbiol 2016 1:16050.
- Chlanda P, Mekhedov E, Waters H, Sodt A, Schwartz C, Nair V, Blank PS, Zimmerberg J. Palmitoylation contributes to membrane curvature in Influenza A virus assembly and hemagglutinin-mediated membrane fusion. J Virol 2017 91:e00947-17.
- Nasamu AS, Glushakova S, Russo I, Vaupel B, Oksman A, Kim AS, Tolia N, Beck JR, Meyers MJ, Niles JC, Zimmerberg J, Goldberg DE. Plasmepsins IX and X are essential and druggable mediators of malaria parasite egress and invasion. Science 2017 358:518–522.
- Glushakova S, Busse BL, Garten M, Beck JR, Fairhurst RM, Goldberg DE, Zimmerberg J. Exploitation of a newly-identified entry pathway into the malaria parasite-infected erythrocyte to inhibit parasite egress. Sci Rep 2017 7:12250.
- Zheng W, Wu Y, Winter P, Fischer R, Nogare DD, Hong A, McCormick C, Christensen R, Dempsey WP, Arnold DB, Zimmerberg J, Chitnis A, Sellers J, Waterman C, Shroff H. Adaptive optics improves multiphoton super-resolution imaging. Nat Methods 2017 14:869-872.
Collaborators
- Oleg Batishchev, PhD, A.N. Frumkin Institute of Physical Chemistry and Electrochemistry, Russian Academy of Sciences, Moscow, Russia
- Josh Beck, PhD, Iowa State University, Ames, IA
- Nikki Curthoys, PhD, University of Maine, Orono, ME
- Rick M. Fairhurst, MD, PhD, Laboratory of Malaria and Vector Research, NIAID, Bethesda, MD
- Vadim Frolov, PhD, Universidad del País Vasco, Bilbao, Spain
- Daniel Goldberg, MD, PhD, Washington University, St. Louis, MO
- Hugo Guerrero-Cazares, MD, The Johns Hopkins University, Baltimore, MD
- Samuel T. Hess, PhD, University of Maine, Orono, ME
- Mary Kraft, PhD, University of Illinois at Urbana-Champaign, Urbana, IL
- Alfredo Quinones-Hinojosa, MD, The Johns Hopkins University, Baltimore, MD
- Thomas S. Reese, MD, Laboratory of Neurobiology, NINDS, Bethesda, MD
- Anna Shnyrova, PhD, Universidad del País Vasco, Bilbao, Spain
- Peter K. Weber, PhD, Lawrence Livermore National Laboratory, Livermore, CA
Contact
For more information, email zimmerbj@mail.nih.gov or visit irp.nih.gov/pi/joshua-zimmerberg.