Biophysics of Large Membrane Channels
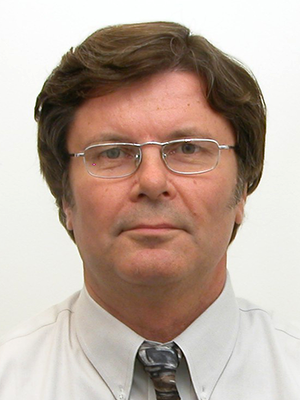
- Sergey M. Bezrukov, PhD, DSci, Head, Section on Molecular Transport
- Tatiana K. Rostovtseva, PhD, Associate Scientist
- Philip A. Gurnev, PhD, Staff Scientist
- Maria Queralt-Martin, PhD, Visiting Fellow
- Amandine Rovini, PhD, Visiting Fellow
Healthy cell functioning and development require both a precise compartmentalization and effective communication between cells and cell organelles. Such processes are critically dependent on the systems regulating metabolism. Specialized membrane channel proteins constitute one such key system that regulates transport of metabolites across the plethora of cell membranes. Deviant alterations in these complex dynamic structures often cause the shift from health to disease. Keeping this in mind, we study “large” beta-barrel channels of eukaryotes and bacteria, which are responsible for metabolite fluxes. The channels are fundamentally different from the conventional ion-selective channels both in their structure and in mechanisms of their selectivity and regulation.
We seek to understand the physical principles of channel functioning under normal and pathological conditions. Among many 'wet-lab' approaches, such as fluorescence correlation spectroscopy and confocal microscopy, our hallmark method is reconstitution of channel-forming proteins into planar lipid membranes, which allows us to study them at the single-molecule level. The combination of experimental work with physical theory gives us the knowledge necessary for intelligent drug design and other strategies to effectively correct aberrant interactions associated with disease.
To grasp the general principles of beta-barrel channel functioning and regulation, we work with a broad variety of channel-forming proteins and peptides. They include the VDAC (Voltage-Dependent Anion Channel from the outer membrane of mitochondria), alpha-Hemolysin (toxin from Staphylococcus aureus), translocation pores of Bacillus anthracis (PA63), Clostridium botulinum (C2IIa), and Clostridium perfringens (Ib) binary toxins, Epsilon toxin (from Clostridium perfringens), OmpF (general bacterial porin from Escherichia coli), LamB (sugar-specific bacterial porin from Escherichia coli), OprF (porin from Pseudomonas aeruginosa), MspA (major outer-membrane porin from Mycobacterium smegmatis), Cytolysin A, (cytolytic pore-forming toxin expressed in virulent strains of Escherichia coli and Salmonella enterica), Alamethicin (amphiphilic peptide toxin from Trichoderma viride), Syringomycin E (lipopeptide toxin from Pseudomonas syringae), and the bacterial peptide TisB involved in persister cell formation. We also use Gramicidin A (linear pentadecapeptide from Bacillus brevis) as a molecular sensor of membrane mechanical properties. With the goal of studying channel-forming proteins under controlled conditions, we first isolate them from the host organisms, purify, and then reconstitute them into planar lipid bilayers, the model membrane system with precisely defined physical properties. This allows us to explore the channels' interactions with the lipid membrane as modified by volatile anesthetics, by cytosolic proteins, such as tubulin and alpha-synuclein, and by newly synthesized drugs that act as blockers of the translocation pores of bacterial toxins. Understanding the molecular mechanisms of channel functioning is vital for developing new approaches to treatment of various diseases, for which aberrations in ion channel transport are implicated in their pathogenesis.
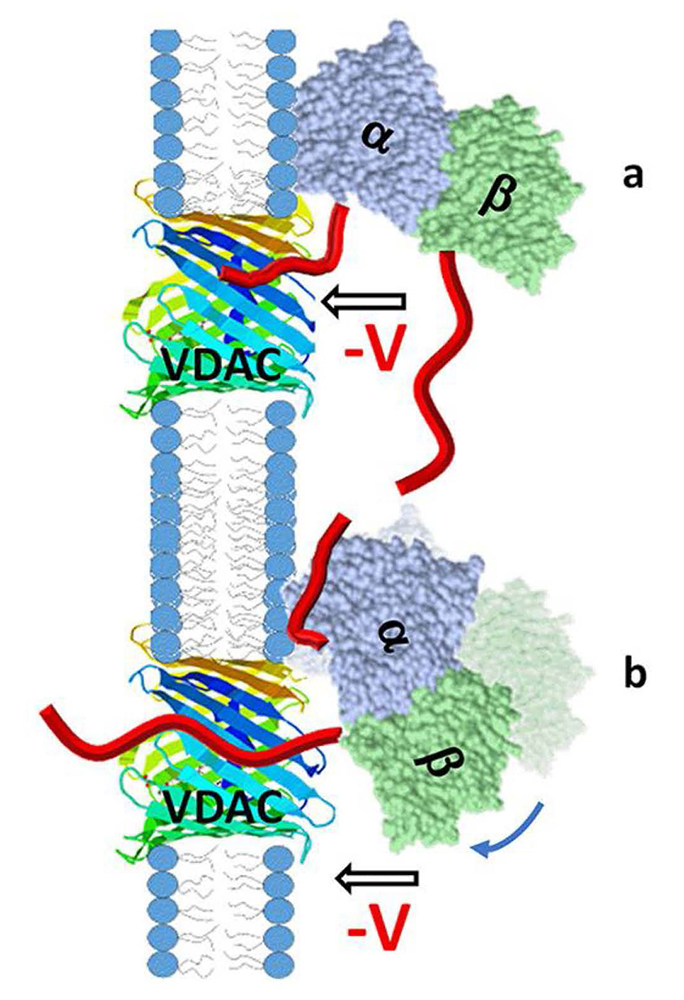
Click image to view.
Figure 1. Cartoon of a model of VDAC pore blockage by the alpha- or beta-CTT of tubulin
The orientation of membrane-bound tubulin dimer was established by us earlier with alpha-tubulin as the membrane-bound subunit. In such alignment, the contribution of the length and especially the first amino acids of the CTT to the blocking kinetics should be different for alpha-CTTs (a) and beta-CTTs (b). There is some degree of rotational dynamics for the membrane-bound alpha-tubulin shown in (b) with the arrow and shadow image, which could also affect the kinetics of pore blockage by the beta-CTT [Reference 1].
Sequence diversity of tubulin isotypes in regulation of mitochondrial respiration
The microtubule protein tubulin is a heterodimer consisting of alpha/beta subunits, each subunit featuring several isotypes in vertebrates. For example, the human proteome contains as many as seven alpha-tubulin and eight beta-tubulin isotypes, which vary mostly in the length and primary sequence of the disordered anionic C-terminal tails (CTTs). The biological reason for such sequence diversity remains a topic of vigorous inquiry. We demonstrated that this diversity may be a key feature of tubulin’s role in regulating the permeability of the mitochondrial outer-membrane voltage-dependent anion channel (VDAC). Previously, we showed that, in addition to their important functions involving interactions with motor proteins and other microtubule-associated proteins, tubulin CTTs are essential for tubulin's interaction with the VDAC. As the major transport channel and most abundant protein in the mitochondrial outer membrane, the channel is responsible for most of the metabolite flux in and out of mitochondria. The VDAC was proven to be involved in a wide variety of mitochondria-associated pathologies, ranging from various forms of cancer to neurodegeneration. This year, using recombinant yeast alpha/beta-tubulin constructs with alpha-CTTs, beta-CTTs, or both from various human tubulin isotypes, we probed their interactions with VDACs reconstituted into planar lipid bilayers. A comparative study of the blockage kinetics revealed that both alpha-CTTs and beta-CTTs block the VDAC pore, but the efficiency of blockage spans two orders of magnitude, depending on the CTT isotype. Beta-tubulin constructs, notably beta3, blocked the VDAC most effectively. We quantitatively described these experimental results, using a physical model that accounts only for the number and distribution of charges in the CTT, and not for the interactions between specific residues on the CTT and VDAC pore. Based on the results, we speculate that the effectiveness of VDAC regulation by tubulin depends on the predominant tubulin isotype in a cell. Consequently, the fluxes of ATP/ADP through the channel could vary significantly depending on the isotype. Our results suggest an intriguing link between VDAC regulation and the diversity of tubulin isotypes present in vertebrates.
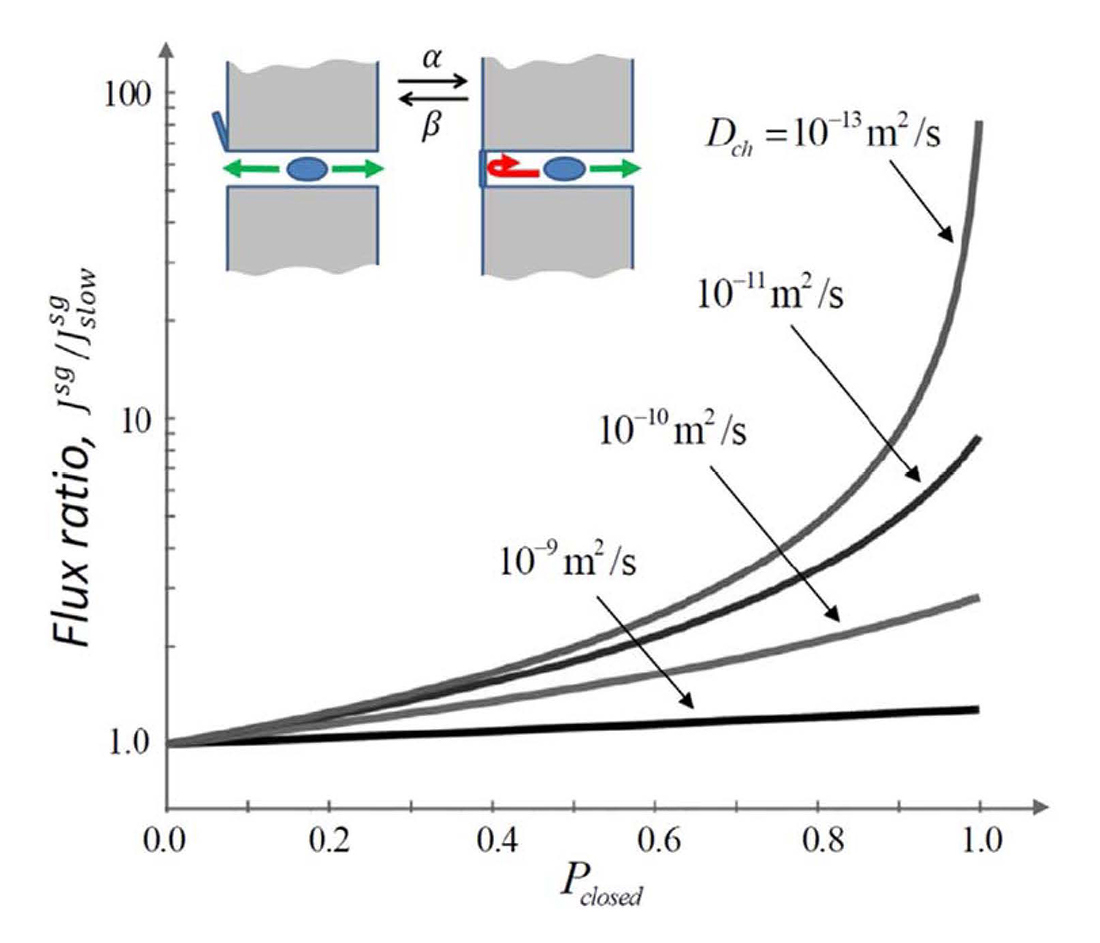
Click image to view.
Figure 2. Flux through a stochastically gated channel
Depending on the molecular intra-channel diffusivity (indicated by the numbers near the curves), the flux through a channel can differ significantly from its conventional estimate. The flux ratio is the ratio of the flux at an arbitrary gating rate to its conventional counterpart [Reference 2].
Stochastic gating as a novel mechanism for channel selectivity
In contrast to the highly ion-selective channels studied in neurophysiology, which have narrow selectivity filters of the size of a partially dehydrated ion, metabolite channels are significantly wider. Indeed, they have to accommodate metabolite molecules that are typically much larger than simple mono- or divalent ions. It is clear that the large pore size of metabolite channels jeopardizes one of the main membrane functions, namely, to serve as a barrier for solutes other than the particular metabolites these channels have evolved to pass. For example, the VDAC, recognized to be the major pathway for ATP and ADP exchange between mitochondria and cytosol, is also highly permeable to molecules smaller than these metabolites. The VDAC is also the most abundant integral protein of the outer mitochondrial membrane. Interestingly however, several studies demonstrated that, under physiological conditions, these channels are predominantly closed, which allows the membrane to sustain its barrier function. This year, by applying our recent theory of the stochastic gating effect on channel-facilitated transport, we proposed that stochastic gating may provide a mechanism for metabolite channel selectivity in favor of slowly moving large solutes. Specifically, we showed that fast gating of a predominantly closed channel leads to an increase, by orders of magnitude, in the channel selectivity for large, slowly diffusing molecules versus small ions. We hypothesized that this, to our knowledge, newly described mechanism of the selectivity attributable to stochastic gating provides an explanation for the puzzling observation that the VDAC in intact mitochondria is mostly closed. Our conjecture is that the large number of predominantly closed VDAC channels are necessary to keep ATP/ADP transport at a sufficiently high level while effectively suppressing small-ion leakage through the mitochondrial outer membrane. It is also worth mentioning that the stochastic gating effect we propose might not be restricted to the VDAC case. We hypothesized that it can be used to minimize the shunting effect of other wide channels with respect to small solutes, thus shedding light on the functioning of various predominantly plugged beta-barrel channels (e.g., the OprF of Pseudomonas aeruginosa) and on other biological processes controlled by passage through fluctuating bottlenecks.
Lipid nanodomains in ion channel functioning
The function of lipid rafts in mammalian cell membranes remains an issue of controversy, though the association of many membrane proteins with the relatively detergent-resistant membrane fraction of plasma membranes has been well established. However, to the best of our knowledge, the ability of nano-sized membrane inhomogeneities to modify channel behavior has not yet been established. This year, using raft-forming model membrane systems containing cholesterol, we showed that lipid lateral phase separation at the nanoscale level directly affects the dissociation kinetics of the gramicidin dimer, a model ion channel. Gramicidin inserts into membranes and dimerizes to form cation-selective ion channels. The conducting lifetime of the channels is exquisitely sensitive not only to lipid composition, but also to compounds that alter membrane mechanics. For a lipid raft model, we studied mixtures of dioleoylphosphatidylcholine, porcine brain sphingomyelin, and cholesterol. We found that the gramicidin channel detects the presence of separate nanoscale domains in the 1/1/1 mixture well above the microscopic miscibility transition, as reported by fluorescence microscopy. Of particular interest for neurophysiology, we noted that the proportion of cholesterol in nerve cell membranes is relatively high, about 40%, and that cholesterol depletion in live mammalian cells inhibits both raft nanodomain formation and activation of the PI(3)K/Akt pathway. Our findings unequivocally demonstrate that nanodomains in cholesterol-rich membranes do affect ion channel function. While the structure of gramicidin indeed differs from that of mammalian ion channels, many of the fundamental principles of ion conduction through channels were first demonstrated with gramicidin, and its sensitivity to the surrounding lipid environment is a property shared by a significant number of ion channels involved in synaptic signaling. Thus, we suggest that perturbations of lipid phase mixing produced by application of membrane-active agents could affect nervous system function through the interactions at the nanoscale that were uncovered in our study.
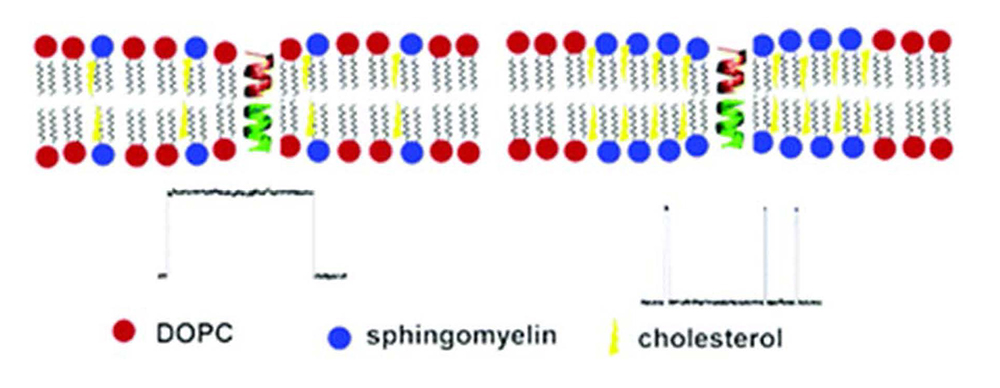
Click image to view.
Figure 3. Cartoon illustrating influence of lipid rafts on ion channels
Lipid lateral phase separation at the nanoscale level directly affects the dissociation kinetics of the gramicidin dimer, a model ion channel. Modulation of channel protein function by the saturated chains and high cholesterol environment in lipid rafts occurs via the changes in the local mechanical properties of the membrane [Reference 3].
Publications
- Rostovtseva TK, Gurnev PA, Hoogerheide DP, Rovini A, Sirajuddin M, Bezrukov SM. Sequence diversity of tubulin isotypes in regulation of the mitochondrial voltage-dependent anion channel. J Biol Chem 2018;293:10949–10962.
- Berezhkovskii AM, Bezrukov SM. Stochastic gating as a novel mechanism for channel selectivity. Biophys J 2018;114:1026–1029.
- Weinrich M, Worcester DL, Bezrukov SM. Lipid nanodomains change ion channel function. Nanoscale 2017;9:13291–13297.
- Hoogerheide DP, Gurnev PA, Rostovtseva TK, Bezrukov SM. Real-time nanopore-based recognition of protein translocation success. Biophys J 2018;114:772–776.
- Berezhkovskii AM, Dagdug L, Bezrukov SM. First passage, looping, and direct transition in expanding and narrowing tubes: effects of the entropy potential. J Chem Phys 2017;147:134104.
Collaborators
- Vicente M. Aguilella, PhD, Universidad Jaume I, Castellón, Spain
- Alexander M. Berezhkovskii, PhD, Division of Computational Bioscience, CIT, NIH, Bethesda, MD
- Susan K. Buchanan, PhD, Laboratory of Molecular Biology, NIDDK, Bethesda, MD
- Leonid V. Chernomordik, PhD, Section on Membrane Biology, NICHD, Bethesda, MD
- Leonardo Dagdug, PhD, Universidad Autónoma Metropolitana-Iztapalapa, Mexico City, Mexico
- David Hoogerheide, PhD, National Institute of Standards and Technology, Gaithersburg, MD
- Jennifer C. Lee, PhD, Biochemistry and Biophysics Center, NHLBI, Bethesda
- Ekaterina M. Nestorovich, PhD, The Catholic University of America, Washington, DC
- Sergei Y. Noskov, PhD, University of Calgary, Calgary, Canada
- Adrian Parsegian, PhD, University of Massachusetts Amherst, Amherst, MA
- Olga Protchenko, PhD, Liver Diseases Branch, NIDDK, Bethesda, MD
- Dan Sackett, PhD, Section on Cell Biophysics, NICHD, Bethesda, MD
- Alexander Sodt, PhD, Unit on Membrane Chemical Physics, NICHD, Bethesda, MD
- Gerhard Wagner, PhD, Harvard Medical School, Cambridge, MA
- Michael Weinrich, MD, Office of the Director, NICHD, Bethesda, MD
- David L. Worcester, PhD, National Institute of Standards and Technology, Gaithersburg, MD
- Joshua Zimmerberg, MD, PhD, Section on Cellular and Membrane Biophysics, NICHD, Bethesda, MD
Contact
For more information, email bezrukov@helix.nih.gov or visit http://smt.nichd.nih.gov.