Molecular Genetics of Heritable Human Disorders
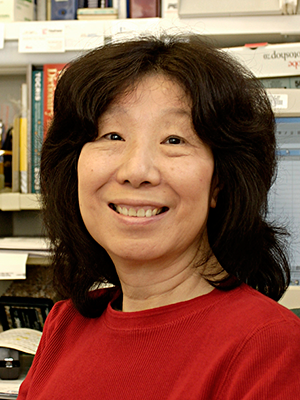
- Janice Y. Chou, PhD, Head, Section on Cellular Differentiation
- Irina Arnaoutova, PhD, Staff Scientist
- Lisa Zhang, PhD, Staff Scientist
- Jun Ho Cho, PhD, Visiting Fellow
- Sudeep Gautam, PhD, Visiting Fellow
- Goo Young Kim, PhD, Visiting Fellow
- Brian C. Mansfield, PhD, Guest Researcher
- Javier Anduaga, BS, Technical Intramural Research Training Award Fellow
We conduct research to delineate the pathophysiology of and develop novel therapies for the three subtypes of type I glycogen storage disease (GSD-I): GSD-Ia, GSD-Ib, and GSD-Irs (GSD-I related syndrome). GSD-Ia is caused by a deficiency in glucose-6-phosphatase-α (G6Pase-α or G6PC), GSD-Ib is caused by a deficiency in the G6P transporter (G6PT or SLC37A4), and GSD-Irs, also known as severe congenital neutropenia syndrome type 4, is caused by a deficiency in G6Pase-β. G6Pase-α and G6Pase-β are endoplasmic reticulum (ER)–bound G6P hydrolases, with active sites lying inside the lumen, which depend on G6PT to translocate G6P from the cytoplasm into the ER lumen. The G6PT/G6Pase-α complex maintains interprandial glucose homeostasis, while the G6PT/G6Pase-β complex maintains energy homeostasis and the functionality of neutrophils. GSD-Ia and GSD-Ib patients manifest a common metabolic phenotype of impaired glucose homeostasis not shared by GSD-Irs, whereas GSD-Ib and GSD-Irs patients manifest a common myeloid phenotype of neutropenia and myeloid dysfunction not shared by GSD-Ia. Inactivation of G6PT or G6Pase-β in neutrophils leads to the enhanced apoptosis that underlies neutropenia in GSD-Ib and GSD-Irs. A deficiency in either G6PT or G6Pase-β in neutrophils prevents recycling of glucose from the ER to the cytoplasm, leading to the impaired energy homeostasis that underlies neutrophil dysfunction in GSD-Ib and GSD-Irs. There is no cure for GSD-Ia, GSD-Ib, or GSD-Irs. Animal models of the three disorders are available and are being exploited to both delineate the diseases more precisely and develop new treatment approaches, including gene therapy. We have generated several efficacious G6Pase-α–expressing recombinant adeno-associated virus (rAAV) vectors and provided a proof-of-principle gene therapy in murine GSD-Ia that is safe, efficacious, and appropriate for entering clinical trials. The rAAV vector expressing a codon-optimized G6Pase-α developed in this laboratory (US patent number: 9,644,216; European patent number: EP3074510) was selected in 2018 Phase I/II clinical trial for human GSD-Ia (ClinicalTrials.gov Identifier: NCT03517085).
Molecular mechanism preventing hepatocellular adenoma and carcinoma (HCA/HCC) in GSD-Ia mice receiving gene therapy
The predominant subtypes of HCA in GSD-Ia are inflammatory HCA (IHCA, 52%) and β-catenin–mutated HCA (bHCA, 28%). We previously showed that non–tumor–bearing (NT), rAAV–treated GSD-Ia mice (AAV-NT mice) expressing a wide range (0.9–63%) of normal hepatic G6Pase-α activity maintain glucose homeostasis and display physiologic features mimicking animals living under calorie restriction. We showed that, in AAV-NT mice, the signaling pathways of the calorie-restriction mediators AMPK and SIRT1 were activated, leading to inhibition of the activity of STAT3 and nuclear factor kappaB (NF-kappaB), pro-inflammatory and cancer-promoting transcription factors. SIRT1 also inhibits cancer metastasis by increasing the expression of E-cadherin, a tumor suppressor, and reducing the expression of mesenchymal markers. Consistently, in AAV-NT mice, hepatic levels of active STAT3 and of the p65 subunit of NF-kappaB were reduced, as were expression of mesenchymal markers, STAT3 targets, NF-kappaB targets, and β-catenin targets. AAV-NT mice also expressed elevated levels of E-cadherin and fibroblast growth factor 21 (FGF21), targets of SIRT1, and β-klotho, which can act as a tumor suppressor. Importantly, treating AAV-NT mice with a SIRT1 inhibitor markedly reversed many of the observed anti-inflammatory/anti-tumorigenic signaling pathways. In summary, activation of hepatic AMPK/SIRT1 and FGF21/β-klotho signaling pathways combined with down-regulation of STAT3/NF-kappaB–mediated inflammatory and tumorigenic signaling pathways can explain the absence of hepatic tumors in AAV-NT mice.
Hepatic G6Pase-α activity is required to prevent HCA/HCC in GSD-Ia.
The hallmarks of GSD-Ia are impaired glucose homeostasis and long-term risk of HCA/HCC. We previously developed a G6Pase-α–expressing rAAV vector, rAAV-G6PC, and showed that rAAV-G6PC–treated G6pc–/– mice expressing 3–63% of normal hepatic G6Pase-α activity (AAV mice) maintain glucose homeostasis and do not develop HCA/HCC. However, the threshold of hepatic G6Pase-α activity required to prevent tumor formation remained unknown. To increase the efficacy of the gene transfer vector, we constructed rAAV-co-G6PC, a rAAV vector expressing a codon-optimized (co) G6Pase-α and showed that rAAV-co-G6PC was more efficacious than rAAV-G6PC in directing hepatic G6Pase-α expression. Over an 88–week study, we showed that both rAAV-G6PC– and rAAV-co-G6PC–treated G6pc–/– mice expressing 3–33% of normal hepatic G6Pase-α activity maintained glucose homeostasis, developed no HCA/HCC, and were protected against age-related obesity and insulin resistance. Of the eleven rAAV-G6PC/rAAV-co-G6PC–treated G6pc–/– mice harboring 0.9–2.4% of normal hepatic G6Pase-α activity (AAV-low mice), three expressing 0.9–1.3% of normal hepatic G6Pase-α activity developed HCA/HCC, while eight did not (AAV-low-NT). We also showed that the AAV-low-NT mice exhibited a phenotype indistinguishable from that of AAV mice expressing 3% or more of normal hepatic G6Pase-α activity. The results establish the threshold of hepatic G6Pase-α activity required to prevent HCA/HCC and show that GSD-Ia mice harboring less than 2% of normal hepatic G6Pase-α activity are at risk for tumor development.
Downregulation of SIRT1 signaling underlies hepatic autophagy impairment in GSD-Ia.
The most severe long-term complication in GSD-Ia is HCA/HCC of unknown etiology. The global G6pc–/– mice die early, well before HCA/HCC can develop, making studies on the mechanism of HCA/HCC in these mice difficult. We therefore generated liver-specific G6pc knock-out (L -G6pc–/–) mice, which survive to adulthood and develop HCA. A recent report showed that G6Pase-α deficiency causes impairment in autophagy, a recycling process important for cellular metabolism. However, the underlying mechanism is unclear. We showed that liver-specific knockout of G6Pase-α led to downregulation of SIRT1 signaling, which activates autophagy via deacetylation of autophagy-related (ATG) proteins, and of the FoxO family of transcriptional factors, which trans-activate autophagy genes. Consistently, defective autophagy in G6Pase-α–deficient liver was characterized by attenuated expressions of autophagy components, increased acetylation of ATG5 and ATG7, decreased conjugation of ATG5 and ATG12, and reduced autophagic flux. We further showed that hepatic G6Pase-α deficiency resulted in activation of ChREBP, a lipogenic transcription factor, increased expression of PPAR-γ, a lipid regulator, and suppressed expression of PPAR-α, a master regulator of fatty acid β-oxidation, all contributing to hepatic steatosis and downregulation of SIRT1 expression. An adenovirus vector–mediated increase in hepatic SIRT1 expression corrected autophagy defects but failed to rectify metabolic abnormalities associated with G6Pase-α deficiency. Importantly, rAAV vector–mediated restoration of hepatic G6Pase-α expression corrected metabolic abnormalities, restored SIRT1-FoxO signaling, and normalized defective autophagy (see Figure). Taken together, the data show that hepatic G6Pase-α deficiency–mediated down-regulation of SIRT1 signaling underlies defective hepatic autophagy in GSD-Ia.
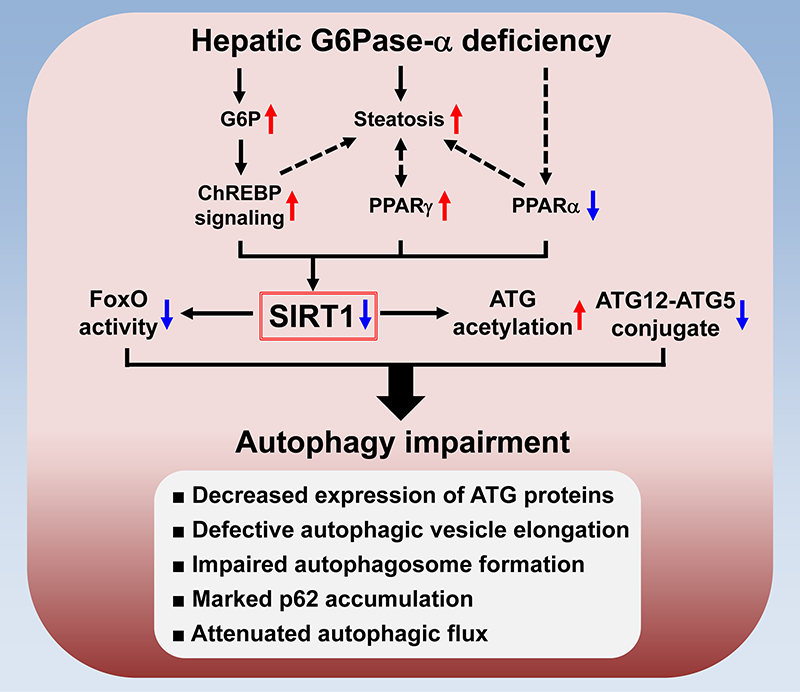
Click image to view.
The mechanism underlying autophagy impairment in hepatic G6Pase-α deficiency
Hepatic G6Pase-α deficiency leads to metabolic alterations including G6P accumulation and suppressed expression of PPARα, a master regulator of fatty acid β-oxidation. The G6P–mediated activation of ChREBP signaling induces lipogenesis, leading to hepatic steatosis, which increases the expression of PPARγ, another lipogenic factor. Moreover, aberrant PPARγ overexpression aggravates hepatic steatosis. The net outcome is downregulation of hepatic SIRT1 signaling. Impaired signaling by SIRT1 increases acetylation of ATG, reduces ATG12–ATG5 conjugation, and down-regulates FoxO signaling, which induces autophagy genes. Accordingly, hepatic G6Pase-α deficiency–mediated autophagy impairment is characterized by reduced expression of ATG proteins, defective autophagic vesicle elongation, impaired autophagosome formation, marked accumulation of the p62 adaptor protein (implicated in selective autophagy), and attenuated autophagic flux.
Liver-directed gene therapy for murine GSD-Ib
The G6pt–/– mice manifest both metabolic and myeloid dysfunction characteristic of human GSD-Ib. When left untreated, the G6pt–/– mice rarely survive weaning, reflecting the juvenile lethality seen in human GSD-Ib patients. Studies have shown that the choice of transgene promoter can impact targeting efficiency, tissue-specific expression, and the level of immune response or tolerance to the therapy. We therefore examined the safety and efficacy of liver-directed gene therapy in G6pt–/– mice using rAAV-GPE-G6PT and rAAV-miGT-G6PT, two G6PT–expressing rAAV8 vectors directed by the human G6PC and G6PT promoter/enhancer, respectively. Both vectors corrected hepatic G6PT deficiency in murine GSD-Ib, but the G6PC promoter/enhancer was more efficacious. Over a 78–week study, we showed that G6pt–/– mice expressing 3–62% of normal hepatic G6PT activity exhibited a normalized liver phenotype. Two of the 12 mice expressing less than 6% of normal hepatic G6PT activity developed HCA. All treated mice were leaner and more sensitive to insulin than wild-type mice. Mice expressing 3–22% of normal hepatic G6PT activity exhibited higher insulin sensitivity than mice expressing 44–62%. The levels of insulin sensitivity correlated with the magnitude of hepatic ChREBP signaling activation. In summary, we established the threshold of hepatic G6PT activity required to prevent tumor formation and showed that mice expressing 3–62% of normal hepatic G6PT activity maintained glucose homeostasis and were protected against age-related obesity and insulin resistance.
Hepatic G6Pase-α deficiency leads to metabolic reprogramming in GSD-Ia.
We had shown that hepatic G6Pase-α deficiency–mediated steatosis leads to defective autophagy that is frequently associated with carcinogenesis, and we recently showed that hepatic G6Pase-α deficiency also leads to enhancement of hepatic glycolysis and hexose monophosphate shunt (HMS), which can contribute to hepatocarcinogenesis. The enhanced hepatic glycolysis is reflected in increased lactate accumulation, increased expression of many glycolytic enzymes, and elevated expression of c-Myc, which stimulates glycolysis. The enhanced HMS is reflected in elevated G6P dehydrogenase activity, elevated production of NADPH, and reduced glutathione. We showed that that restoration of hepatic G6Pase-α expression normalizes both glycolysis and HMS in GSD-Ia. Moreover, the HCA/HCC lesions in L-G6pc–/– mice exhibit elevated levels of hexokinase 2 and the M2 isoform of pyruvate kinase, which play an important role in aerobic glycolysis and cancer cell proliferation. Taken together, hepatic G6Pase-α deficiency causes metabolic reprogramming, leading to enhanced glycolysis and elevated HMS, which, along with impaired autophagy, can contribute to HCA/HCC development in GSD-Ia.
Additional Funding
- The Children's Fund for Glycogen Storage Disease Research, 2017
- Dimension Therapeutics (Cambridge, MA) under a Cooperative Research and Development Agreement (CRADA)
Publications
- Kim GY, Lee YM, Kwon JH, Cho JH, Mansfield BC, Chou JY. Downregulation of pathways implicated in liver inflammation and tumorigenesis of glycogen storage disease type Ia mice receiving gene therapy. Hum Mol Genet 2017;26:1890-1899.
- Cho J-H, Kim GY, Pan CJ, Anduaga J, Choi E-J, Mansfield BC, Chou JY. Downregulation of SIRT1 signaling underlies hepatic autophagy impairment in glycogen storage disease type Ia. PLoS Genet 2017;13:e1006819.
- Kim GY, Lee YM, Kwon JH, Cho JH, Pan CJ, Starost MF, Mansfield BC, Chou JY. Glycogen storage disease type Ia mice with less than 2% of normal hepatic glucose-6-phosphatase-alpha activity restored are at risk of developing hepatic tumors. Mol Genet Metab 2017;120:229-234.
- Kwon JH, Lee YM, Cho J-H, Kim GY, Anduaga, J, Starost MF, Mansfield BC, Chou JY. Liver-directed gene therapy for murine glycogen storage disease type Ib. Hum Mol Genet 2017;26(22):4395-4405.
- Cho JH, Kim GY, Mansfield BC, Chou JY. Sirtuin signaling controls mitochondrial function in glycogen storage disease type Ia. J Inherit Metab Dis 2018;Epub ahead of print.
Collaborators
- Eui-Ju Choi, PhD, Korea University, Seoul, South Korea
- Alessandra Eva, PhD, Istituto Giannina Gaslini, Genova, Italy
- Hyun Sik Jun, PhD, Korea University, Seoul, South Korea
- Youngmok Lee, PhD, University of Connecticut School of Medicine, Farmington, CT
- David A. Weinstein, MD, MSc, University of Connecticut School of Medicine, Farmington, CT
Contact
For more information, email chou@helix.nih.gov or visit https://irp.nih.gov/pi/janice-chou.