Gene Regulation in Innate Immunity

- Keiko Ozato, PhD, Head, Section on Molecular Genetics of Immunity
- Anup Dey, PhD, Biologist
- Tiyun Wu, PhD, Staff Scientist
- Mahesh Bachu, PhD, Visiting Fellow
- Sakshi Chauhan, PhD, Visiting Fellow
- Chao Chen, PhD, Visiting Fellow
- Eri Hosogane, PhD, Visiting Fellow
- Vishal Nehru, PhD, Visiting Fellow
- Keita Saeki, MD, PhD, Visiting Fellow
- Sumihito Togi, PhD, Visiting Fellow
- Richard Pan, BS, Postbaccalaureate Fellow
Macrophages and related cells such as microglia recognize incoming pathogens and produce cytokines, notably interferons (IFNs), the interleukins IL-1 and IL-6, and tumor necrosis factor alpha (TNF-alpha). While IFNs impart anti-viral and anti-microbial protection to the host, the latter cytokines are associated with inflammatory responses. IFNs are produced upon activation of the IRF (interferon regulatory factor) family of transcription factors, while inflammatory cytokines are produced by activation of the transcription factor NFκB. Our goal is to study the molecular pathways that direct the development and function of macrophages and other myeloid cells. To this end, we focus on the role of IRF8 in innate immunity. IRF8, a member of the IRF family, is expressed at high levels in macrophages, microglia, and dendritic cells (DCs) and is required for the production of both type I and type II IFNs. IRF8 is essential for mounting the first line of defense against various invading pathogens prior to the initiation of antigen-specific adaptive immune responses.
Transcriptionally active genes are embedded in chromatin that is dynamically exchanged, whereas silenced genes are surrounded by more stable chromatin. The chromatin environment contributes to the epigenetic states of given cells and influences transcriptional processes. We have long been working on BRD4, a bromodomain protein that binds to acetylated histones and promotes active transcription. BRD4 is involved in the dynamic chromatin exchange that takes place in highly transcribed genes, an exchange that requires a special histone called H3.3. As a result of the association with transcription, H3.3 is implicated in epigenetic control of gene expression patterns. Our goal is to elucidate the activity of BRD4 and histone H3.3 in innate immunity.
The role of IRF8 in brain inflammation: Alzheimer's disease and Aicardi-Goutières syndrome
IRF8 is a transcription factor of the conserved interferon regulatory factor family. It is expressed in cells important for host anti-pathogen protection and is involved in inflammatory responses. Recent evidence shows that, through its expression in microglia, IRF8 plays a significant role in inflammation in the brain. SNP–based genome-wide association studies revealed that inflammatory genes expressed in microglia are risk factors for Alzheimer's disease (AD), which causes memory loss followed by breakdown of broader cognitive function. We have begun to study the role of IRF8 in AD using the mouse model of AD. Histological studies found that microglia scattered over the entire brain have abnormal morphology in IRF8 knockout (KO) mice (Figure 1). IRF8 KO microglia were all devoid of extensive dendrites projecting next to neurons and appeared similar to those in AD models. RNA-seq analysis of wild-type (WT) and IRF8 KO microglia showed that a large array of AD–associated genes were activated in IRF8 KO microglia (Figure 2). Some of the genes (red dots) were found to be AD risk factors, including ApoE (encoding apolipoprotein E), which represented the highest risk factor.
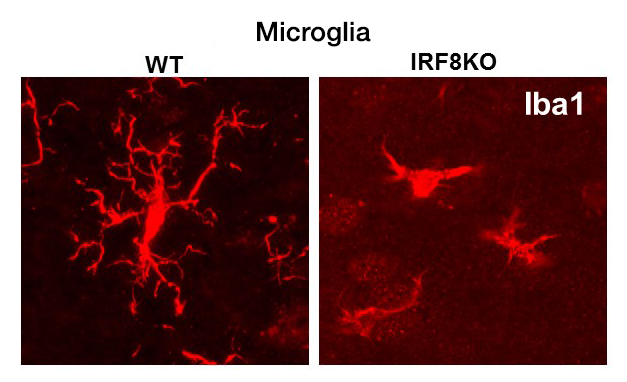
Click image to view.
Figure 1. Microglia in IRF8 KO brain
Immunohistochemical analysis of microglia in wild-type and IRF8 KO (knockout) brain (one-month-old). Microglia were stained with the microglia-specific marker Iba1.
Aicardi-Goutières syndrome (AGS) patients present with varying forms of encephalopathy with prominent neuroinflammation. Symptoms include vision/motor deficits, and cognitive deficiency and vascular damage in the brain associated with lupus (SLE)–like autoimmune conditions. Classically, AGS has been linked to mutations in enzymes that degrade endogenous nucleic acids (DNA and RNA), produced by normal biological processes, including replication, transcription, and DNA repair [Crow YJ, Manel N. Nat Rev Immunol 2015;15:429]. Mutations in any of the three RNase H2 subunits account for those in more than 50% of AGS patients. Mutations in other nucleic acid–metabolizing factors linked to AGS are in TREX1 (encoding three-prime repair exonuclease 1), ADAR (encoding double-stranded RNA-specific adenosine deaminase), and SAMHD1 (encoding deoxynucleoside triphosphate triphosphohydrolase). More recently, constitutively activating mutations in the signaling pathways that sense excess nucleic acids and activate innate immune responses have been found to also cause AGS–like diseases. The Crouch lab showed that defects in RNase H2 lead to accumulation of DNA and activate the cGAS-STING pathway, which detects the presence of cytosolic DNA; loss of Sting eliminates activation of interferon-stimulated genes (ISGs). Accumulation of RNA triggers the RIG-I/MDA5 pathway, which plays a major role in sensing RNA-virus infection by detecting cytoplasmic viral double-stranded RNA. Both pathways signal Tbk1 (a serine/threonine kinase that plays an essential role in regulating inflammatory responses to foreign agents), leading to activation of type I interferons and their downstream pathways. Thus, activation of ISGs, coined the "interferon signature," is a defining feature of AGS. ISG proteins are found in spinal fluids, presumably produced in microglia (although not proven), and in peripheral monocytes/macrophages. Recent developments in the field have broadened the description of AGS with regard to its onset, phenotypic severity, and variability. Therefore, in collaboration with Robert Crouch and Yoh-suke Mukouyama, we are studying how IRF8 affects microglial inflammatory responses in mice carrying the mutation in the RNAseH2A, which causes AGS in humans.
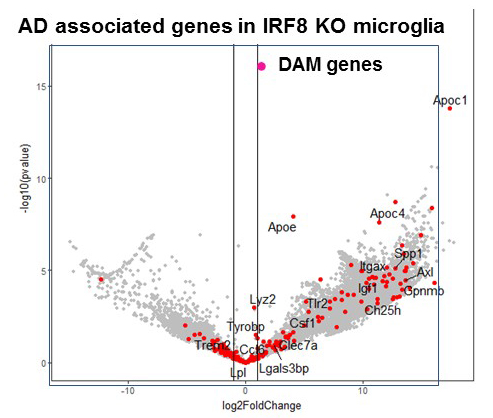
Click image to view.
Figure 2. Alzheimer's disease–associated genes in IRF8 KO microglia
Microglia were sorted from wild-type and IRF8 KO brain (3-month-old), and RNA seq (an RNA sequencing method) analyses were performed. Genes upregulated in IRF8 KO microglia were enriched with genes upregulated in microglia of the Alzheimer's disease mouse model.
Hematopoietic stem cells depend on BRD4 for their maintenance and progenitor differentiation.
BRD4 is a bromodomain protein of the BET (bromodomain and extraterminal domain) family, which this laboratory has been studying for many years. BRD4 is expressed at high levels in most, if not all cells, and is necessary for very early embryonic development. Thus, conventionally created Brd4 knockout (KO) mice are embryonic lethal. BRD4 is a so-called "chromatin reader" owing to its binding to acetylated histones. It also recruits the transcription elongation factor P-TEFb, thus facilitating transcriptional elongation. Moreover, BRD4 has a critical role in forming super-enhancers, long stretches of regulatory DNAs densely occupied by transcription factors and chromatin regulators. The super-enhancers direct strong transcription of select genes and thus help define cellular and lineage identity. In the past several years, research on BRD4 has seen a dramatic upturn owing to the development of small-molecule inhibitors that inhibit binding of acetyl-histones to the BET family proteins. The inhibitors, affecting mostly BRD4, antagonize cancer growth, particularly leukemia and lymphoma. Furthermore, BET inhibitors have been shown to inhibit inflammatory responses related to cardiovascular and autoimmune diseases. Such reports implicate BRD4 in various disease processes and offer new therapeutic possibilities for several difficult-to-treat illnesses; indeed, clinical trials are being conducted for leukemia and inflammation. However, the developments present new issues stemming from the dearth of our understanding of the precise role of BRD4 in health and disease and of the mechanism of BRD4 action. Studies on inhibitors have inherent limitations owing to uncertainty regarding their specificity, modality of action, and long-term consequences. For example, the impact of BET inhibitors on normal hematopoietic cells is not well understood, posing potential problems when treating blood cancers such as leukemia/lymphoma. BET inhibitor treatment may compromise the activity and maintenance of hematopoietic stem cells and may weaken the ability to combat infection, which is also relevant to treating inflammation, given that macrophages are the main effector of both inflammation and host defense.
We thus sought to gain a fuller understanding of BRD4's activity in normal hematopoiesis and during inflammatory and innate immune responses. We studied Brd4 conditional knockout (KO) mice, focusing on hematopoiesis and macrophage responses. First, we tested mice in which Brd4 is deleted in early hematopoiesis using the Vav-Cre technique. We showed that Brd4 KO mice die during fetal development as a result of severe defects in the expansion of hematopoietic stem cells (HSC) and in the development of hematopoietic progenitor cells. As a consequence, Brd4 KO embryos fail to develop immune cells of all lineages, including lymphocytes and myeloid cells, which are important for innate and adaptive immunity. We also found that BRD4 is essential for the proliferation of macrophages, based on LysM-Cre–dependent deletion of Brd4 (LysM-Cre selectively targets macrophages and neutrophils); the resultant Brd4 KO mice failed to start IL-4–dependent peritoneal macrophage expansion. The results strongly point to a central role of BRD4 in immune cell expansion, which is required for maintaining immunity.
We investigated genome-wide distribution of BRD4 in macrophages in a resting condition and after lipopolysaccharide (LPS) stimulation. LPS is a pathogen component that rapidly induces inflammatory genes and interferon-stimulated genes important for protection against pathogens. We found that BRD4 broadly occupies genic and intergenic regions. Within the genic region, BRD4 binding peaked at the transcription start site (TSS), although binding was detected over the 5′ promoter and within coding regions. BRD4 binding over genic regions markedly increased after LPS stimulation, indicating that BRD4 moves rapidly over the genome, presumably to accommodate a rapid alteration of histone acetylation.
Because it has been proposed that BRD4 is central to the generation of super-enhancers, we were interested to know whether Brd4 KO macrophages possessed super-enhancers. We found that BRD4 displayed dense clustering over distant regulatory regions that represented super-enhancers. BRD4 clusters coincided with the H3K27 chromatin mark, which denotes super-enhancers as well as RNA polymerase II clustering. BRD4–containing super-enhancers localize to genes important for basic macrophage phenotypes and innate immune responses.
NSD2 guides interferon-induced H3.3 deposition and regulates transcription by recruiting the chromatin reader ZMYND.
IFNs rapidly stimulate transcription of many IFN–stimulated genes (ISGs). We previously showed that ISG transcription is coupled with H3K36 trimethylation and deposition of the histone variant H3.3, creating stable epigenetic marks on ISGs. NSD2 (WHSC1, MMSET1) is a histone methyltransferase that methylates H3K36. We showed that global distribution and IFN–induced deposition of H3.3 was abrogated in mouse embryonic fibroblasts from Nsd2 KO mice, with only minor changes in the H3K36me3 distribution pattern, leading to aberrant ISG induction, in that a fraction of ISGs were constitutively expressed in Nsd2 KO cells even prior to IFN stimulation. Further, more than half the ISGs (about 200) were induced more rapidly and at higher levels in KO cells than in wild-type cells. ZMYND11 is a "chromatin reader" that was recently reported to recognize H3.3 and shown to repress transcription of some genes. We demonstrated that ZMYND11 is recruited to ISGs upon IFN stimulation and that recruitment is inhibited in Nsd2 KO cells. Consistent with these data, exaggerated ISG expression was observed in ZMYND11 KO cells. Our results reveal that ISG induction is internally coupled to NSD2-ZMYND11–dependent transcriptional suppression, which restrains transcriptional overdrive. ZMYND11–mediated repression may be linked to epigenetic memory for ISGs.
Additional Funding
- NICHD Scientific Director's Award (2018-2019)
Publications
- Kamada R, Yang W, Xhang Y, Patel M, Yang Y, Wakabayashi Y, Ouda R, Sakagichi K, Fujita T, Tamura T, Zhu, J, Ozato K. Interferon stimulation creates heritable chromatin marks to establish transcriptional memory. Proc Natl Acad Sci USA 2018;115(39):E9162-E9171.
- Haque N, Ouda R, Ozato, K, Hogg JR. Zinc finger RNA-binding protein controls IFNbeta transcription through histone variant macroH2A1. Nat Commun 2018;20;9(1):1145.
- Ouda R, Sarai N, Nehru V, Patel MC, Debrosse M, Bachu M, Chereji RV, Eriksson PR, Clark DJ, Ozato K. SPT6 interacts with NSD2 and facilitates interferon-induced transcription. FEBS Lett 2018;592:1681-1692.
- Yu X, Chen H, Zuo C, Jin X, Yin Y, Wang H, Jin M, Ozato K, Xu S. Chromatin remodeling: demethylating H3K4me3 of type I IFNs gene by Rbp2 through interacting with Piasy for transcriptional attenuation. FASEB J 2018;32:552-567.
- Cougnoux A, Drummond RA, Collar AL, Iben JR, Salman A, Westgarth H, Wassif CA, Cawley NX, Farhat NY, Ozato K, Lionakis MS, Porter FD. Microglia activation in Niemann-Pick disease, type C1 is amendable to therapeutic intervention. Hum Mol Genet 2018;15(27):2076-2089.
Collaborators
- Robert J. Crouch, PhD, Section on Formation of RNA, NICHD, Bethesda, MD
- Steven Holland, MD, Laboratory of Clinical Infectious Diseases, NIAID, Bethesda, MD
- Katrin D. Mayer-Barber, Dr rer nat, Laboratory of Clinical Infectious Diseases, NIAID, Bethesda, MD
- Herbert Morse II, MD, Laboratory of Immunopathology, NIAID, Rockville, MD
- Yoh-suke Mukouyama, PhD, Laboratory of Stem Cell and Neuro-Vascular Biology, NHLBI, Bethesda, MD
- Dinah S. Singer, PhD, Experimental Immunology Branch, NCI, Bethesda, MD
- Tomohiko Tamura, MD, PhD, Tokyo University, Tokyo, Japan
- Jun Zhu, PhD, DNA Sequencing and Genomics Core, NHLBI, Bethesda, MD
Contact
For more information, email ozatok@mail.nih.gov or visit http://ozatolab.nichd.nih.gov.