Olfactory Coding and Decoding by Neuron Ensembles
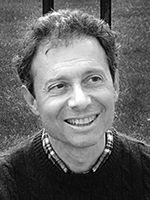
- Mark Stopfer, PhD, Head, Section on Sensory Coding and Neural Ensembles
- Zane Aldworth, PhD, Staff Scientist
- Alejandra Boronat Garcia, PhD, Postdoctoral Fellow
- Yu-Shan Hung, PhD, Postdoctoral Fellow
- Subhasis Ray, PhD, Postdoctoral Fellow
- Bo-Mi Song, PhD, Postdoctoral Fellow
- Kui Sun, MD, Technician
- Brian Kim, BS, Postbaccalaureate Fellow
Oscillatory integration windows in neurons
Oscillatory synchronization of neurons occurs in many brain regions, including the olfactory systems of vertebrates and invertebrates, and is indispensable for precise olfactory coding. One mechanism by which oscillations have been proposed to influence coding is through the creation of cyclic integration windows—specific times within the oscillation cycle when synaptic input is most efficiently integrated by a post-synaptic neuron. Cyclic integration windows could allow a neuron to respond preferentially to spikes arriving coincidentally from multiple presynaptic neurons in a specific part of the cycle. Thus, coincidence detection mediated by integration windows could help read precise temporal codes for odors. Phase-specific effects of synaptic inputs have been described in both brain slices and simulations. However, the existence of cyclic integration windows has not been demonstrated, and their functional requirements are unknown.
With paired local field potential (LFP) and intracellular recordings and controlled stimulus manipulations, we directly tested this idea in the locust olfactory system. We focused on the responses of Kenyon cells, which are high-order neurons in a brain area analogous to the vertebrate piriform cortex and which fire spikes when the animal is presented with an odor pulse. We found that inputs arriving in Kenyon cells sum most effectively in a preferred window of the oscillation cycle. With a computational model, we established that the non-uniform structure of noisy activity in the membrane potential helps mediate this process. Further experiments performed in vivo demonstrated that integration windows can form in the absence of inhibition and at a broad range of oscillation frequencies.
Our results establish that cyclic integration windows can be formed from very few ingredients: oscillatory input and noise in the membrane potential. Given the ubiquity of membrane noise, the mechanisms we describe likely apply to a wide variety of neurons that receive oscillatory inputs, with or without inhibition and across a range of frequencies. Our results reveal how a fundamental coincidence-detection mechanism in a neural circuit functions to decode temporally organized spiking.
Spatio-temporal coding of individual chemicals by the gustatory system
Four of the five major sensory systems (vision, olfaction, somatosensation, and audition) are thought to be encoded by spatio-temporal patterns of neural activity. The only exception is gustation. Gustatory coding by the nervous system is thought to be relatively simple: every chemical ('tastant') is associated with one of a small number of basic tastes, and the presence of a basic taste, rather than the specific tastant, is represented by the brain. In mammals as well as insects, five basic tastes are usually recognized: sweet, salty, sour, bitter, and umami. The neural mechanism for representing basic tastes is unclear. The most widely accepted postulate is that, in both mammals and insects, gustatory information is carried through labelled lines—that is, in separate channels from the periphery to sites deep in the brain—of cells sensitive to a single basic taste. An alternative proposal is that the basic tastes are represented by populations of cells, with each cell sensitive to several basic tastes.
Testing these ideas requires determining, point-to-point, how tastes are initially represented within the population of receptor cells and how this representation is transformed as it moves to higher-order neurons. However, it has been highly challenging to deliver precisely timed tastants while recording cellular activity from directly connected cells at successive layers of the gustatory system. Using a new moth preparation, we designed a stimulus and recording system that allowed us to fully characterize the timing of tastant delivery and the dynamics of the tastant-elicited responses of gustatory receptor neurons and their mono-synaptically connected second-order gustatory neurons, before, during, and after tastant delivery.
Surprisingly, we found no evidence consistent with a basic taste model of gustation. Instead, we found that the moth’s gustatory system represents individual tastant chemicals as spatio-temporal patterns of activity distributed across the population of gustatory receptor neurons. We further found that the representations are transformed substantially, given that many types of gustatory receptor neurons converge broadly upon follower neurons. The results of our physiological and behavioral experiments suggest that the gustatory system encodes information not about basic taste categories but rather about the identities of individual tastants. Furthermore, the information is carried not by labelled lines but rather by distributed, spatio-temporal activity, which is a fast and accurate code. The results provide a dramatically new view of taste processing.
A population of projection neurons that inhibits the lateral horn but excites the antennal lobe through chemical synapses in Drosophila
The insect antennal lobe is a useful model system in which to study neural computations. Drosophila has been a particularly beneficial model system because it offers numerous genetic tools for labeling and manipulating the activity of neurons. In the insect olfactory system, odor information is transferred from the antennal lobe to higher brain areas by projection neurons running through many antennal lobe tracts. In several species, one of these tracts, the mediolateral antennal lobe tract (mlALT), contains projection neurons expressing GABA, a neurotransmitter that usually elicits inhibition; in the Drosophila brain, the great majority of ventral projection neurons (vPNs) are GABAergic and project through the tract to a brain area called the lateral horn. Most projection neurons, which are excitatory (ePNs), project through the mALT to the lateral horn and to another brain area, the mushroom body. Recent studies showed that GABAergic vPNs play inhibitory roles at their axon terminals in the lateral horn. However, little is known about the properties and functions of vPNs at their dendritic branches in the antennal lobe.
We used genetic manipulations and optogenetic and patch-clamp techniques to investigate the functional roles of vPNs in the antennal lobe. Surprisingly, our results show that specific activation of vPNs always elicits strong excitatory post-synaptic potentials in ePNs, even though most vPNs are GABAergic. Moreover, we found that the connections between vPNs and ePNs are mediated by direct chemical synapses rather than, as has been previously reported, by gap junctions. Neither pulses of GABA nor pharmacological or genetic blockade of GABAergic transmission gave results consistent with the involvement of GABA in vPN–ePN excitatory transmission. A possibility we cannot rule out is that GABAergic vPNs co-express an excitatory neurotransmitter and release it at specific compartments within cells; for example, GABA could be released at the axonal terminals in the lateral horn and an excitatory neurotransmitter released at the dendritic presynaptic terminals in the antennal lobe. Indeed, several examples of mammalian neurons that can release multiple fast excitatory or inhibitory neurotransmitters have been reported, such as spatially segregated release of GABA and ACh in the retina. These unexpected results suggest new roles for the vPN population in olfactory information processing.
Publications
- Gupta N, Singh SS, Stopfer M. Oscillatory integration windows in neurons. Nat Commun 2016;7:13808.
- Reiter S, Campillo Rodriguez C, Sun K, Stopfer M. Spatiotemporal coding of individual chemicals by the gustatory system. J Neurosci 2015;35:12309-12321.
- Boronat-García A, Reiter S, Sun K, Stopfer M. New methods to study gustatory coding. J Vis Exp 2017;124:e55868.
- Shimizu K, Stopfer M. A population of projection neurons that inhibits the lateral horn but excites the antennal lobe through chemical synapses in Drosophila. Front Neural Circuits 2017;11:30.
- Alvarez-Prats A, Bjelobaba I, Aldworth Z, Takashi B, Abebe D, Kim YJ, Stojilkovic SS, Stopfer M, Balla T. Schwann-cell-specific deletion of phosphatidylinositol 4-kinase alpha causes aberrant myelination. Cell Rep 2018;23(10):2881-2890.
Collaborators
- Tamás Balla, MD, PhD, Section on Molecular Signal Transduction, NICHD, Bethesda, MD
- Maxim Bazhenov, PhD, Howard Hughes Medical Institute, The Salk Institute for Biological Studies, La Jolla, CA
Contact
For more information, email stopferm@mail.nih.gov or visit https://neuroscience.nih.gov/Faculty/Profile/mark-stopfer.aspx or https://irp.nih.gov/pi/mark-stopfer.