Hippocampal Interneurons and Their Role in the Control of Network Excitability
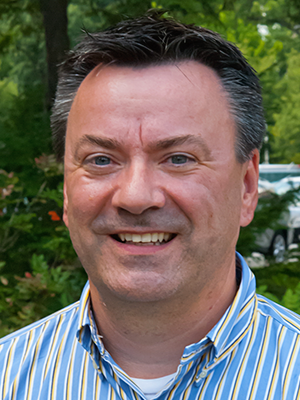
- Chris J. McBain, PhD, Head, Section on Cellular and Synaptic Physiology
- Ramesh Chittajulla, PhD, Staff Scientist
- Kenneth Pelkey, PhD, Staff Scientist
- Gulcan Akgul, PhD, Postdoctoral Fellow
- James D'Amour, PhD, Postdoctoral Fellow
- Vivek Madahavan, PhD, Postdoctoral Fellow
- Jason Wester, PhD, Postdoctoral Fellow
- Steven Hunt, Biologist
- Xiaoqing Yuan, MSc, Biologist
- Tyler Ekins, BSc, Predoctoral Fellow
- Carolina Bengtsson, Predoctoral Fellow
Neocortical projection neurons instruct inhibitory interneuron circuit development in a lineage dependent manner.
Neocortical circuits, for all their complexity, demonstrate a stereotypical organization across regions, with many repeated motifs, which suggests there may be general principles that guide the self-assembly of these circuits during development. A particularly interesting problem for the developing cortex is how to integrate inhibitory interneurons (INs), which are produced by progenitors outside the telencephalon, into circuits among locally generated excitatory projection neurons (PNs). Upon reaching the nascent cortex, INs must coordinate with their new host environment to establish proper positioning, wiring, and total numbers. There is accumulating evidence that this process involves subtype-specific interactions between INs and neighboring PNs. Investigation of the process is daunting, given the large diversity among INs; however, it is possible that the interactions are organized within primary classes of PNs and INs. Recent evidence suggests that the subtype identity of both PNs and INs is important for the process. We knocked out the transcription factor Satb2 in PNs to induce those of the intratelencephalic (IT) type to adopt a pyramidal tract (PT) identity. Loss of IT PNs disrupted the lamination and molecular expression profile of INs derived selectively from caudal ganglionic eminence (CGE), and in a subtype-specific manner. Reprogrammed PNs demonstrated altered connectivity with local CGE INs, with a striking reduction in synaptic targeting of all subtypes. In deep layers of control mice, where IT and PT PNs are intermingled, IT PNs targeted neighboring CGE INs, while PT PNs did not, confirming this lineage-dependent motif. Our data show that both PN class and IN embryonic lineage are important general variables during the construction of cortical circuits.
Subunits of the glutamate receptor AMPAR differentially regulate cellular and synaptic maturation of CGE–derived hippocampal interneurons.
Hippocampal GABAergic local circuit INs target distinct domains of their postsynaptic targets to gate incoming excitatory input, control firing of PNs, and pace synchronized activity among neurons in both neonatal and adult brain. To date more than 20 subgroups of GABAergic INs have been identified in the rodent CA1 hippocampus. Fate mapping strategies revealed that distinct interneuron subtypes are derived from either the medial ganglionic eminence (MGE) precursor pools (e.g. parvalbumin [PV]–, somatostatin [SOM]–, and neuronal nitric oxide synthase [nNOS]–containing interneurons) or from CGE precursor pools (e.g., cholecystokinin [CCK]–, vasoactive intestinal polypeptide [VIP]–, reelin [RE]– and calretinin [CR]–containing interneurons). On exiting proliferation, interneurons migrate tangentially towards the hippocampus using both genetic [Pleasure et al., Neuron 2000;28:727] and environmental cues. Cell numbers are then consolidated through programmed apoptotic cell death that, in part, relies on activity-dependent regulation through a calcineurin-dependent mechanism. INs regulate all aspects of hippocampal circuit activity, and their dysregulation may contribute to numerous neural-circuit disorders. An understanding of how interneurons are integrated into the developing hippocampal circuit is crucial for establishing future therapeutic strategies. We showed that elimination of AMPAR–mediated excitatory input onto CGE–derived interneurons during development alters emerging anatomical, synaptic, and circuit properties, resulting in deficits in social interaction and hippocampal-dependent learning.
In the hippocampal CA1 microcircuit, CGE–derived INs are recruited by activation of glutamatergic synapses comprising GluA2–containing calcium-impermeable AMPARs. However, the role played by AMPARs in cell migration, survival, and maturation of the developing circuit is unknown. We generated two knockout mouse lines in which we selectively eliminated Gria2 (GluA2 KO), Gria1, Gria2, and Gria3 (GluA1–3 KO), genes that encode subtypes of ionotropic glutamate receptors, in 5HT3AR–Cre–expressing CGE–derived interneurons (5HT3AR is an ionotropic serotonin receptor on a subtype of GABAergic interneurons). In both neonatal (P5–9) and juvenile (P17–21) hippocampus spontaneous excitatory postsynaptic currents (sEPSC), the frequency onto CGE interneurons was significantly reduced in the GluA2 KO, which was coupled with a reduction in dendritic glutamatergic synapse density. Elimination of GluA1–3 almost completely eliminated sEPSCs without further reducing synapse density, but increased the complexity of dendritic branching. In GluA1–3 KOs, the number of interneurons invading the hippocampus was increased in the early postnatal period but converged with wild-type (WT) numbers by P21 due to an increased apoptosis. However, GluA1–3 KOs showed an increase in CCK–containing and a reduction in VIP–containing interneurons. Elimination of GluA1–3–containing synapses significantly reduced recruitment of both feedforward and feedback inhibitory input onto pyramidal neurons and altered the contribution of MGE– versus CGE–derived interneuron-mediated inhibition. As a consequence of these combined anatomical, synaptic, and circuit alterations, GluA2 KO and GluA1–KO mice exhibited impaired learning and deficits in social behavior. Thus, AMPAR subunits differentially contribute to numerous aspects of the development and maturation of CGE–derived interneurons and hippocampal circuitry that are essential for normal animal behavior.
Molecular dissection of Neuroligin2 and Slitrk3 reveals an essential framework for GABAergic synapse development.
Synapse development is a multi-step process orchestrated by numerous molecules acting in a highly spatially and temporally controlled manner. Among these molecules, synaptic cell adhesion molecules (CAMs) are a class of membrane proteins essential for the establishment and maturation of synaptic connections. Recent studies identified a growing number of synaptic CAMs, including Neuroligins (NLs) and leucine-rich repeat transmembrane proteins (LRRTMs) that bind to presynaptic neurexins, Slit– and Trk–like family proteins (Slitrks) that bind to presynaptic protein tyrosine phosphatases (PTPs), immunoglobulin superfamily proteins (IgSFs) that mediate trans-synaptic homophilic or heterophilic adhesion interactions, cadherin family proteins, and transmembrane tyrosine kinase receptors. Although these molecules are involved in various stages of synapse development, how diverse synaptic CAMs work in concert to control synapse formation remains largely unclear.
In the brain, many types of interneurons make functionally diverse inhibitory synapses onto principal neurons. Although numerous molecules have been identified that function in inhibitory synapse development, it remains unknown whether there is a unifying mechanism for the development of diverse inhibitory synapses. We reported a general molecular mechanism underlying hippocampal inhibitory synapse development. In developing neurons, the establishment of GABAergic transmission depends on Neuroligin 2 (NL2), a synaptic CAM. During maturation, inhibitory synapse development requires both NL2 and Slitrk3 (ST3), another CAM. Importantly, NL2 and ST3 interact with nanomolar affinity through their extracellular domains to synergistically promote synapse development. Selective perturbation of the NL2–ST3 interaction impairs inhibitory synapse development, with consequent disruptions in hippocampal network activity and increased seizure susceptibility. Our findings reveal how unique postsynaptic CAMs work in concert to control synaptogenesis and establish a general framework for GABAergic synapse development.
Additional Funding
- Jason Wester was funded by an NINDS Intramural National Research Service Award (NRSA)
- James D’Amour was funded by an NIGMS PRAT Fellowship
Publications
- Wester J, McBain CJ. Inhibitory interneurons differentially contribute to spontaneous network activity in the developing hippocampus dependent on their embryonic lineage. J Neurosci 2016;36:2646-2662.
- Calvigioni D, Máté Z, Fuzik J, Girach F, Zhang M-D, Varro A, Beiersdorf J, Schwindling C, Yanagawa Y, Dockray G, McBain CJ, Hokfelt T, Szabó G, Keimpema E, Harkany T. Functional differentiation of cholecystokinin-containing interneurons destined for the cerebral cortex. Cereb Cortex 2017;27:2453-2468.
- Chittajallu R, Wester JC, Craig MT, Barksdale E, Yuan X, Akgul G, Fang C, Collins D, Hunt S, Pelkey KA, McBain CJ. Afferent specific role of NMDA receptors for the circuit integration of hippocampal neurogliaform cells. Nat Commun 2017;8(1):152.
- Li J, Han W, Pelkey KA, Duan J, Mao X, Wang Y-X, Dong L, Craig MT, Petralia RS, McBain CJ, Lu W. Molecular dissection of Neuroligin2 and Slitrk3 reveals an essential framework for GABAergic synapse development. Neuron 2017;96:808-826.
- Pelkey KA, Chittajallu R, Craig MT, Tricoire L, Wester JC, McBain CJ. Hippocampal GABAergic inhibitory interneurons. Physiol Rev 2017;97:1619-1747.
Collaborators
- Wei Lu, PhD, Synapse and Neural Circuit Unit, NINDS, Bethesda, MD
Contact
For more information, email mcbainc@mail.nih.gov or visit https://neuroscience.nih.gov/Faculty/Profile/chris-mcbain.aspx.