Building the Zebrafish Lateral Line System
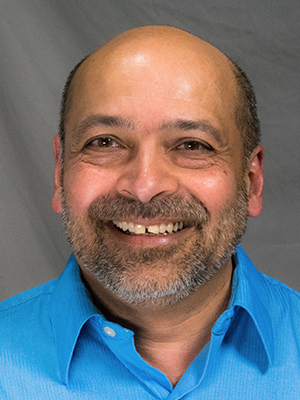
- Ajay Chitnis, MBBS, PhD, Head, Section on Neural Developmental Dynamics
- Damian E. Dalle Nogare, PhD, Staff Scientist
- Gregory Palardy, BS, Research Technician
- Chongmin Wang, MS, Research Technician
- Pritesh Krishnakumar, PhD, Postdoctoral Fellow
- Uma Neelathi, PhD, Postdoctoral Fellow
- Jon Schrope, BS, Postbaccalaureate Intramural Research Training Award Fellow
Cells divide, move, adhere, and interact with their neighbors and their environment to determine the formation of multicellular organ systems with unique fates, morphologies, function, and behavior. Our goal is to understand how such interactions determine the self-organization of cell communities in the nervous system of the zebrafish embryo. The lateral line is a mechano-sensory system that helps sense the pattern of water flow over the fish and amphibian body and consists of sensory organs called neuromasts that are distributed in a stereotypic pattern over the body surface. Each neuromast has sensory hair cells at its center, surrounded by support cells, which serve as progenitors for production of more hair cells during growth and regeneration of neuromasts. The development of this superficial sensory system in zebrafish is spearheaded by the posterior lateral line primordia, groups of about 150 cells formed on either side of a day-old embryo near the ear. Cells in the primordia migrate collectively under the skin to the tip of the tail, as they divide and reorganize to form nascent neuromasts that are deposited sequentially from its trailing end. Their journey is easily observed in live transgenic embryos with fluorescent primordium cells. Furthermore, a range of genetic and cellular manipulations can be used to investigate gene function and morphogenesis in the system. Understanding the self-organization of this relatively simple and accessible system in zebrafish will help elucidate the broader principles that determine cell fate specification, morphogenesis, and collective cell migration in the developing vertebrate nervous system.
Self-organization of the zebrafish lateral line primordium
Formation of the posterior lateral line system in zebrafish is pioneered by the posterior Lateral Line (pLL) primordium, a group of about 150 cells that forms near the ear. While leading cells in the pLL primordium have a relatively mesenchymal morphology, trailing cells are more epithelial; they have distinct apical/basal polarity and reorganize to sequentially form nascent neuromasts or protoneuromasts. The pLL primordium begins migration toward the tip of the tail at about 22 hours post fertilization (hpf). Proliferation adds to the growth of the primordium; nevertheless, as the primordium migrates, the length of the column of cells undergoing collective migration progressively shrinks, as cells stop migrating are deposited from the trailing end. Thus, cells that were incorporated into protoneuromasts are deposited as neuromasts, while cells that were not, are deposited between neuromasts as interneuromast cells. Eventually, the primordium ends its migration a day later after depositing 5–6 neuromasts and by resolving into 2–3 terminal neuromasts.
The Wnts (Wingless/Integrated) and Fgfs (fibroblast growth factors) are evolutionarily conserved secreted proteins that allow cells to communicate with their neighbors via distinct signaling pathways to influence various aspects of their neighbor's behavior, fate, shape and capacity to proliferate. Wnt and Fgf signaling systems coordinate morphogenesis and migration of the primordium. Thus, Wnt signaling dominates at the leading end and is thought to determine the relatively mesenchymal morphology of leading cells, while Fgf signaling dominates in the trailing end. There, Fgf determines reorganization of groups of trailing cells to form rosettes, as the cells constrict at their apical ends. Furthermore, Fgf signaling determines the specification of a central cell in each rosette as a sensory hair cell progenitor and helps determine collective migration of the pLL primordium cells. Wnt signaling promotes its own activity and at the same time drives expression of fgf3 and fgf10. However, leading cells do not respond to these Fgf ligands because Wnt signaling simultaneously promotes expression of intracellular inhibitors of the Fgf receptor. Instead, the Fgfs activate Fgf receptors and initiate Fgf signaling at the trailing end of the primordium, where Wnt signaling is weakest. There, Fgf signaling determines expression of the diffusible Wnt antagonist Dkk1b, which counteracts Wnt signaling to help establish stable Fgf–responsive centers. Once established, the trailing Fgf signaling system coordinates morphogenesis of nascent neuromasts by simultaneously promoting the reorganization of cells into epithelial rosettes and by initiating expression of factors that help specify a sensory hair cell progenitor at the center of each forming neuromast. Over time, the leading domain with active Wnt signaling shrinks closer to the leading edge, and additional Fgf signaling centers form sequentially in its wake, each associated with formation of additional protoneuromasts.
The interactions between the leading Wnt system and the trailing Fgf system provide a useful framework for understanding the self-organization of neuromast formation and deposition by the migrating pLL primordium; however, many questions remain unanswered. The Wnt and Fgf signaling systems act simply as a means of communication between cells, and it remains unclear what molecular mechanisms the systems regulate to specifically determine morphogenesis of epithelial rosettes and the collective migration of primordium cells. Furthermore, the mechanics of collective migration in the primordium remains poorly understood, specifically, how the pull of leading cells, which migrate in response to chemokine cues in their path, determines the Fgf–dependent collective migration of trailing cells in the primordium. The summary above suggests that morphogenesis of epithelial rosettes during the assembly of nascent neuromasts is entirely dependent on Fgf signaling. However, it has been observed that, in the absence of collective migration mediated by chemokines in the leading cells, the trailing cells in the primordium come together to form one or two large rosettes. These and other observations related to the changes in the number and size of epithelial rosettes in the presence and absence of collective migration suggest that primordium cells have an inherent potential to form epithelial rosettes and that the formation of rosettes can be influenced by a variety of signaling systems and/or by migratory behavior of leading cells. Our attention has now shifted to answering some of the questions outlined here.
Cxcl12a induces snail1b expression to initiate collective migration and sequential Fgf–dependent neuromast formation in the zebrafish pLL primordium.
Our discovery that snail1b is expressed in leading cells led us to speculate that its expression might be determined by Wnt signaling and that, as a factor known for its role in promoting epithelial-mesenchymal transition (EMT), it might determine mesenchymal morphology of leading cells. However, our analysis revealed that Wnt signaling does not determine snail1b expression in the leading zone. Instead, its expression in the leading zone is promoted by chemokine signals first encountered by leading cells of the primordium, while Fgf signaling prevents snail1b expression in trailing cells. The effect of knocking down snail1b function was also not what we expected. It did not compromise the ability of leading cells to adopt a mesenchymal morphology. Instead, sequential morphogenesis of epithelial rosettes associated with formation of protoneuromasts in the trailing domain was delayed in snail1b morphants. Our analysis revealed, however, that the delay in protoneuromast formation is not related to a specific role of Snail1b in morphogenesis of epithelial rosettes. Instead, it is indirectly related to the role of Snail1b in helping initiate effective collective migration of the primordium, a role consistent with its previously described role in promoting cell movement. Interestingly, we found that other manipulations that prevent effective primordium migration also cause a similar delay in sequential formation of Fgf signaling centers and associated protoneuromasts and a shrinking of the leading Wnt system. Taken together, these observations reveal an unexpected role for collective migration of the primordium in kick-starting sequential formation of additional protoneuromasts. We also showed that problems in initiating collective migration in the primordium may, at least in part, be related to aberrant expansion of the cell-adhesion molecule Epcam into the leading zone and/or the loss of cdh2 expression from the leading zone of the primordium following knock-down of snail1b function.
Characterizing cell migration, proliferation, lineage, and fate specification in the migrating pLL primordium at single-cell resolution
Many basic aspects of the biology of the pLL primordium, including proliferation patterns, lineage relationships, and the dynamics of neuromast deposition remain incompletely characterized. To unambiguously resolve such questions, we conducted time-lapse imaging of the pLL primordium, first at low resolution for some embryos, and then again for three independent embryos at a resolution that allowed us to track and catalog every single cell in the pLL primordium from early in migration until termination (approximately 24 hours). From this dataset, we constructed digital models of the pLL primordium. We could query the models to define the lineages of specific cells or subsets of cells, such as those that form specific neuromasts or that adopt specific fates within the neuromasts. Previous studies had suggested that the leading zone with Wnt activity maintains a proliferative population of progenitor cells that divide to produce a subset of daughter cells that move to a trailing zone, where, under the influence of Fgf signaling, they differentiate to sequentially form neuromasts. However, our analysis revealed that proliferation in the pLL primordium is largely unpatterned and not especially high in the leading zone. Furthermore, protoneuromasts are not formed in the trailing zone from a self-renewing population of progenitors in a leading zone. Instead, they are formed by the local proliferation of cells along the length of the primordium in response to Fgf signaling, as the Wnt signaling system, which locally inhibits protoneuromast formation, is progressively restricted to a smaller leading zone of the pLL primordium. The fate of deposited cells as central sensory hair cell progenitors, within neuromasts, surrounding support cells, or as interneuromast cells between deposited neuromasts, is not determined by any obvious stereotyped lineages. Instead, their fate is determined, somewhat stochastically, as a function of a cell’s distance from the center of a maturing protoneuromast. This observation is consistent with the notion that a cell’s fate is determined in the pLL primordium by its level of exposure to active Fgf signaling; the central cell in the protoneuromast, most likely specified earlier as a sensory hair cell progenitor by its exposure to the highest level of Fgf signaling and via lateral inhibition mediated by Notch, becomes a source of Fgf 10. We suggest that proximity to this new central source of Fgfs subsequently determines the level of Fgf signaling and the eventual fate of surrounding cells in the maturing protoneuromast.
Publications
- Neelathi UM, Dalle Nogare D, Chitnis AB. Cxcl12a induces snail1b expression to initiate collective migration and sequential Fgf-dependent neuromast formation in the zebrafish posterior lateral line primordium. Development 2018;145(14):dev162453.
Collaborators
- Hari Shroff, PhD, Laboratory of Molecular Imaging and Nanomedicine, NIBIB, Bethesda, MD
Contact
For more information, email chitnisa@mail.nih.gov or visit https://irp.nih.gov/pi/ajay-chitnis.