Global Regulation of Gene Expression by ppGpp
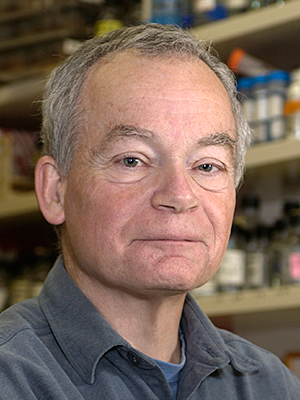
- Michael Cashel, MD, PhD, Head, Section on Molecular Regulation
- Llorenc Fernandez-Coll, PhD, Postdoctoral Intramural Research Training Award Fellow
- Krishma M. Tailor, PhD, Volunteer
Our continuing research goal is to understand the emerging powerful regulatory functions of compounds that are closely related to the four common precursor ribonucleotides (GTP, ATP, UTP, and CTP) and that are polymerized into RNA. RNA is a polymer of ribose (R) linked by a phosphate bonded at its two (3′ and 5′) sites […pR(pR)pR…] with the bases G, A, U, or C bases attached to the ribose. Unlike the precursors, this family of analogs are all blocked from polymerization because their 3′ and 5′ ribose sites are already bonded to one or more phosphate. We focus on one subset of this family of analogs that have both ribose sites blocked by 3′ and 5′ polyphosphates (ppGpp and pppGpp [here termed (p)ppGpp]). For the other family subset, one ribose has its 3′ and 5′ sites attached to single phosphate to make a cyclic bond (cAMP, cGMP). Other examples are mononucleotides whose two phosphates similarly block each other’s 3′ and 5′ sites (c-di-AMP, c-di-GMP, c-di-AG). Both (p)ppGpp and cAMP were discovered about a half century ago, and both fields are currently very active with discoveries of new analogs and new regulatory functions. Cyclic regulators are biologically ubiquitous; the ppGpp–like regulators are found in bacteria and plant chloroplasts but not in animals, making them a particularly appealing target for new antibiotics.
We focus on bacterial (p)ppGpp for several reasons: the regulatory effects in the model E. coli are global, owing to direct RNA polymerase binding, and can provide targets for antibiotic development that will not affect humans. The (p)ppGpp nucleotides sense a variety of nutritional and physical sources of stress that impair growth. They then accumulate to warn cells of stress and respond with an astonishing array of regulatory functions that allow survival. Many mechanisms are known that protect pathogens from host defenses to thereby aggravate pathogenicity. Evidence for the fundamental role of (p)ppGpp in pathogenicity is pervasive, both with respect to antibiotic resistance and the reduction of host carrier states. The current crisis occasioned by the lack of new antibiotics coupled with the emergence of “superbugs” has led to widespread interest in (p)ppGpp. Our lab is too small to undertake pharmaceutical development. Instead, we focus on ppGpp–like compounds in the hope that a fundamental understanding will continue to underlie practical advances in this field.
ppGpp affects carbohydrate-dependent growth transitions, but in a different manner than cAMP.
When cells grow exponentially on a mixture of glucose and lactose, they first exhaust the glucose, then growth lags transiently because adjustments of gene expression are needed before growth can resume on lactose. The growth pause between serial use of two sugars is termed a “diauxic” lag. Early research on cAMP revealed that it represses the utilization of lactose until glucose is exhausted and subsequently discovered that AMP interacts with lac-operon promoter DNA to turn off lactose usage in preference to glucose. The relative abundance of (p)ppGpp is known to affect this process. Levels of ppGpp are determined by the balance of the activities of two synthetases and one hydrolase. We compared diauxic lags in wild-type cells with mutants of one or another ppGpp synthetase under many conditions, including controls that bypassed cAMP effects, and directly measured (p)ppGpp levels. Our efforts suggest a surprising explanation. Acetate is measurably formed and excreted during growth on glucose, only to be reabsorbed later and, in the presence of (p)ppGpp, converted to acetylphosphate (Ac-P). This pathway is the reverse of the normal pathway for making Ac-P from acetyl-CoA. Ac-P is a high-energy donor for post-translational acetylation of a large number of proteins that alter activities of glycolysis and the Krebs cycle. Enhanced protein acetylation was confirmed during the lag by Western plots with anti-acetyl-lysine monoclonal antibody. We also found that basal ppGpp levels correlate directly with basal Ac-P levels. While details of the mechanism underlying this correlation remain to be uncovered, this reveals a new level of regulatory complexity, i.e., global transcriptional regulation by ppGpp appears to intersect with regulation by a known post-translational regulator, Ac-P [Reference 5].
Phage T4 transcription during infection is reduced without dksA but not without ppGpp.
When in our lab, former postdoctoral fellow Tamara James observed that the plaque sizes of wild-type E. coli infected with phage T4 were larger than those of infected E. coli deleted for the RNA polymerase–binding transcription factor dksA or for ppGpp (ppGppo). This is of interest given that ppGpp and DksA both often act directly on RNA polymerase (RNAP). The 20–minute time course of the T4 infection cycle consists of early, middle, and late transcripts. Early promoters use unmodified RNA polymerase, and early transcripts do not terminate but instead read through middle promoters. Middle transcripts are made with a modified RNAP, which recruits two early phage phiX proteins, MotA and AsiA. Late promoters use a RNAP additionally modified by three middle proteins. Biological measures during infection included a new semi-automated statistical method devised to document plaque size populations along with classical estimates of burst sizes and latent periods. The NICHD Sequencing Core performed RNA-seq analysis complemented by our RT-qPCR and primer extension analyses. The effect of ΔdksA is to double burst size without changing the timing of phage release and to increase early and some middle transcripts by readthrough. The increased middle gene transcripts appear to partially compensate for RNAP modification defects for mutant phage with a partly active MotA protein. In vitro assays of early phage phiX promoter transcripts with RNA polymerase revealed that DksA did not inhibit activity, which suggests that the effects of deletions in vivo are indirect. Surprisingly, ppGppo had only modest effects on all parameters measured, despite statistical documentation of enlarged plaques. Direct ppGpp assays might address the possibility that infection quickly abolishes the ppGpp accumulation response. The work has already attracted attention because it may provide a way to enhance the yield of phage phiX surface vectors loaded with therapeutic payloads with cell-specific targets. This is the first study of effects of DksA and ppGpp on lytic bacterial phage development [Reference 1].
ppGpp alters RNAP structure to enhance DksA inhibition of rrnB P1 promoter initiation.
A key element for triggering the global stringent regulatory response is inhibition of ribosomal RNA synthesis at ribosomal promoters. Two distinct ppGpp binding sites on RNAP sites are known from our work and from that of others. Site 1 is located on a boundary of the large β′ subunit and the smallest ω subunit; Δomega mutants are viable with modest effects on the stringent response. Site 2 is 60Å away from site 1 and formed only in the presence of DksA binding; ΔDksA adversely alters rrnB P1 inhibition and the stringent response. In collaboration with the Murakami laboratory, we soaked preformed RNAP holoenzyme crystals with DksA alone or with the added presence of ppGpp, which allowed structural documentation of conformational changes due to each addition. Bound DksA has access to both the template strand at the active site and a downstream DNA site. Addition of ppGpp modifies this complex in a manner that explains its ability to inhibit rrnB P1 initiation by collapsing open complexes before they can initiate RNA synthesis and forming the first diester bond. Our laboratory showed that cellular ppGpp is a more potent inhibitor than pppGpp and that site 1 binds to both ppGpp and pppGpp. Similar studies might reveal whether different affinities of the two isomers for site 2 might account for this behavior. These observations provide definitive structural evidence for allosteric effects of ppGpp and DksA on transcription regulation [Reference 2].
Opposing effects of (p)ppApp and (p)ppGpp on RNA polymerase structure and function
As mentioned in earlier annual reports, while a postdoctoral our lab, Katarzyna Potrykus discovered a substrate-specificity difference between a eukaryotic (p)ppGpp “Mesh” hydrolase and the E.coli and Streptococcal equisimilis hydrolases. The bacterial hydrolases cleave (p)ppGpp but not (p)ppApp, while the Mesh enzyme cleaves both. The most recent step in our continuing collaboration the Potrykus lab compared (p)ppApp isomers and (p)ppGpp isomers with respect to effects on the standard rrnB P1 promoter activity and, in collaboration with the Murakami laboratory, also identified new RNA polymerase binding sites. The adenine analogs were demonstrated to activate the standard rrnB P1 promoter transcripts. Regulatory features of the adenine analogs on transcription were assessed and found to be more modest than those of (p)pGpp and, surprisingly, were often the opposite of those known for (p)ppGpp. Three examples are: (1) (p)ppApp activates initiation of transcription, whereas (p)ppGpp inhibits; (2) pppApp is a more potent activator than ppApp, whereas ppGpp is more potent inhibitor than pppGpp; and (3) pppApp activation of transcription stabilizes open complexes, whereas ppGpp destabilizes open complexes. Evidently, a 'yin-yang' regulatory relationship exists between pppApp and ppGpp.
Order-of-addition experiments reveal that the analog added first dominates the regulatory outcome over the regulatory effect of the one added later and vice versa. The findings suggest different binding sites for the G and A analogs as well as a high degree of plasticity of RNAP conformational changes, seemingly each kinetically stable enough that, once formed, are able to persist, despite the added presence of the second analog. Soaking pppApp into RNA holoenzyme crystals reveals that pppApp binds to an entirely new site located near the catalytic polymerization site near the switch region. The new site is distinct from the DksA–ppGpp binding site 2 and distinct from ppGpp binding site 1. Moreover, the placement of the new pppApp binding site is consistent with its ability to stabilize open complexes. RNA polymerase gel shifts, detected by electromobility shift assay (EMSA) and occasioned by additions of pppApp and ppGpp, and order of addition experiments are also consistent with regulatory effects. Many years ago, (p)ppApp was reported to accumulate in extracts of Bacillus subtilis, a soil bacterium. The comparison of (p)ppApp and (p)ppGpp analogs suggests that, during evolution, RNA polymerase evolved to harbor a (p)ppApp–specific binding site, which could imply a widespread biological occurrence of (p)ppApp. Future experiments will be aimed at searching for cellular (p)ppApp [Reference 3].
Publications
- Patterson-West J, James TD, Fernández-Coll L, Iben JR, Moon K, Knipling L, Cashel M, Hinton DM. The E. coli global regulator DksA reduces transcription during T4 infection. Viruses 2018;6:308.
- Molodtsov V, Sineva E, Zhang L, Huang X, Cashel M, Ades SE, Murakami KS. Allosteric effector ppGpp potentiates the inhibition of transcript initiation by DksA. Mol Cell 2018;69:828-839.
- Bruhn-Olszewska B, Molodtsov V, Sobala, M, Dylewski M, Murakami KS, Cashel M, Potrykus K. Structure-function comparisons of (p)ppApp vs (p)ppGpp for Escherichia coli RNA polymerase binding sites and for rrnB P1 promoter regulatory responses in vitro. Biochim Biophys Acta Gene Regul Mech 2018;186:731-742.
- Potrykus K, Cashel M. Growth at the best and worst of times. Nat Microbiol 2018;3:862-863.
- Fernandez-Coll L, Cashel M. Contributions of SpoT hydrolase, SpoT synthetase, and RelA synthetase to carbon source diauxic growth transitions in Escherichia coli. Front Microbiol 2018;9:1802.
Collaborators
- Deborah Hinton, PhD, Gene Expression and Regulation Section, NIAID, Bethesda, MD
- Katsuhiko S. Murakami, PhD, The Huck Institutes of the Life Sciences, Penn State University, University Park, PA
- Katarzyna Potrykus, PhD, University of Gdansk, Gdansk, Poland
Contact
For more information, email cashel@mail.nih.gov or visit http://smr.nichd.nih.gov.