The Molecular Mechanics of Eukaryotic Translation Initiation
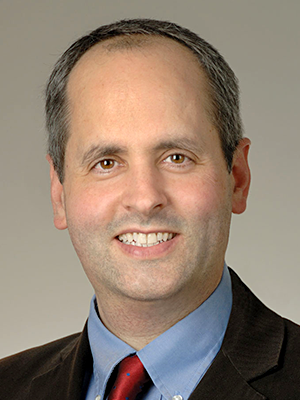
- Jon Lorsch, PhD, Chief, Laboratory on the Mechanism and Regulation of Protein Synthesis
- Jagpreet Nanda, PhD, Staff Scientist
- Fujun Zhou, PhD, Research Fellow
- Shardul Kulkarni, PhD, Postdoctoral Intramural Research Training Award Fellow
- Kaitlynn M. Donahue, MS, Postbaccalaureate Intramural Research Training Award Fellow
The goal of our research group is to elucidate the molecular mechanisms underlying the initiation phase of protein synthesis in eukaryotic organisms. We use the yeast Saccharomyces cerevisiae as a model system and employ a range of approaches—from genetics to biochemistry to structural biology—in collaboration with Alan Hinnebusch's and Tom Dever’s labs and several other research groups around the world.
Eukaryotic translation initiation is a key control point in the regulation of gene expression. It begins when an initiator methionyl tRNA (Met-tRNAi) is loaded onto the small (40S) ribosomal subunit. Met-tRNAi binds to the 40S subunit as a ternary complex (TC) with the GTP–bound form of the initiation factor eIF2. Three other factors, eIF1, eIF1A, and eIF3, also bind to the 40S subunit and promote the loading of the TC. The resulting 43S pre-initiation complex (PIC) is then loaded onto the 5′ end of an mRNA with the aid of eIF3 and the eIF4 group of factors: the RNA helicase eIF4A; the 5′ 7-methylguanosine cap-binding protein eIF4E; the scaffolding protein eIF4G; and the 40S subunit– and RNA–binding protein eIF4B. Both eIF4A and eIF4E bind to eIF4G and form the eIF4F complex. Once loaded onto the mRNA, the 43S PIC is thought to scan the mRNA in search of an AUG start codon. The process is ATP–dependent and likely requires several RNA helicases, including the DEAD–box protein Ded1p. Recognition of the start site begins with base pairing between the anticodon of tRNAi and the AUG codon. Base pairing then triggers downstream events that commit the PIC to continuing initiation from that point on the mRNA, events that include ejection of eIF1 from its binding site on the 40S subunit, movement of the C-terminal tail (CTT) of eIF1A, and release of phosphate from eIF2, which converts eIF2 to its GDP–bound state. In addition, the initiator tRNA moves from a position that is not fully engaged in the ribosomal P site [termed P(OUT)] to one that is [P(IN)], and the PIC as a whole converts from an open conformation that is conducive to scanning to a closed one that is not. At this stage, eIF2•GDP dissociates from the PIC, and eIF1A and a second GTPase factor, eIF5B, coordinate joining of the large ribosomal subunit to form the 80S initiation complex. In a process that appears to result in conformational reorganization of the complex, eIF5B hydrolyzes GTP and then dissociates along with eIF1A.
The molecular mechanics of eukaryotic translation initiation
Our work on the DEAD-box RNA helicase eIF4A was published last year [Reference 1], as was work on the mechanistic consequences of mutations in eIF1A that are associated with uveal melanoma [Reference 2]. We completed two additional manuscripts in fiscal year (FY) 2018. One describes the cryo-electron microscopy structure of a yeast 48S pre-initiation complex in which eIF1 has departed following start codon recognition and has been replaced by the N-terminal domain of eIF5. Biochemical analyses done in our lab of variants of eIF5 made based on this new structure strongly support its mechanistic relevance as a key intermediate in the start codon recognition pathway. The work was done in close collaboration with the labs of Venki Ramakrishnan and Adesh Saini [Reference 3]. In studies on the yeast DEAD-box RNA helicase Ded1, we provided evidence that Ded1 acts as part of a Ded1-eIF4G-eIF4A-eIF4E tetrameric complex to unwind structures in the 5′ UTRs of mRNAs that inhibit PIC attachment and scanning. We also showed that different mRNAs engage with this Ded1 complex in distinct ways, leading to multiple, mRNA–dependent mechanisms for Ded1 function [Reference 4].
We continued our genome-wide analysis of the effects of growth temperature on start codon usage. The work showed that translation of some, but not all, upstream open reading frames (uORFs) is modulated by growth temperature, but in different ways that depend on the specific mRNA. Most regulated uORFs starting with AUG behave similarly, with their translation repressed at low temperature and activated at higher temperature, whereas uORFs starting with near-cognate codons do not show coherent behavior but can be activated, repressed, or remain unchanged at lowered or elevated temperatures. We spent a considerable amount of effort this year looking into specific cases of altered uORF translation that might have physiological consequences, work that should set the stage for future studies, once the initial manuscript is published.
We also made progress on our studies of genome-wide translation initiation using our fully reconstituted yeast-based in vitro system. Using poly(dT)–purified mRNA from yeast, we can examine the initiation efficiency on each mRNA by isolating 48S complexes assembled in vitro, using sucrose gradient ultracentrifugation, followed by RNase footprinting, library construction, and deep sequencing. The power of the in vitro system lies in our ability to manipulate components in ways that are impossible in vivo and to isolate only the direct effects on translation initiation. For example, we can omit components or use lethal variants of factors and determine the effect on initiation for every message simultaneously.
We also initiated a mutational analysis to determine the mechanistic roles of each residue in the N-terminal tails (NTTs) of eIF1 and eIF5. The factors have coordinated but antagonistic functions in the initiation process, and their unstructured NTTs have been shown to be important; however, their exact roles remain largely undefined. We are systematically mutating each residue in the NTTs of these factors and determining the phenotypic effects in vivo in yeast. Variants with strong phenotypes will then be analyzed in the in vitro reconstituted system to define the effects of the changes on key steps in the initiation process.
Additional Funding
- Funding is provided via a Memorandum of Understanding (MOU) between NIGMS and NICHD.
Publications
- Yourik P, Aitken CE, Zhou F, Gupta N, Hinnebusch AG, Lorsch JR. Yeast eIF4A enhances recruitment of mRNAs regardless of their structural complexity. Elife 2017;6:e31476.
- Martin-Marcos P, Zhou F, Karunasiri C, Zhang F, Dong J, Nanda J, Kulkarni SD, Sen ND, Tamame M, Zeschnigk M, Lorsch JR, Hinnebusch AG. eIF1A residues implicated in cancer stabilize translation preinitiation complexes and favor suboptimal initiation sites in yeast. Elife 2017;6:e31250.
- Llácer JL, Hussain T, Saini AK, Nanda JS, Kaur S, Gordiyenko Y, Kumar R, Hinnebusch AG, Lorsch JR, Ramakrishnan V. Translational initiation factor eIF5 replaces eIF1 on the 40S ribosomal subunit to promote start-codon recognition. Elife 2018;7:e39273.
- Gupta N, Lorsch JR, Hinnebusch AG. Yeast Ded1 promotes 48S translation pre-initiation complex assembly in an mRNA-specific and eIF4F-dependent manner. Elife 2018;7:e38892.
Collaborators
- Thomas Dever, PhD, Section on Protein Biosynthesis, NICHD, Bethesda, MD
- Alan Hinnebusch, PhD, Section on Nutrient Control of Gene Expression, NICHD, Bethesda, MD
- Nicholas Ingolia, PhD, University of California at Berkeley, Berkeley, CA
- Venkatraman Ramakrishnan, PhD, MRC Laboratory of Molecular Biology, Cambridge, United Kingdom
- Adesh Saini, PhD, Shoolini University, Solan, India
- Leoš S. Valášek, PhD, Institute of Microbiology, Czech Academy of Sciences, Prague, Czech Republic
Contact
For more information, email jon.lorsch@nih.gov or visit https://irp.nih.gov/pi/jon-lorsch.