Activity-Dependent Regulation of Neurons by the Neuregulin-ErbB Signaling Pathway

- Andres Buonanno, PhD, Head, Section on Molecular Neurobiology
- Detlef Vullhorst, PhD, Staff Scientist
- Irina Karavanova, PhD, Research Assistant
- Sonu Singh, PhD, Visiting Fellow
- Miguel Skirzewski, PhD, Visiting Fellow
- Eric Starr, PhD, Postdoctoral Intramural Research Training Award Fellow
- Larissa Erben, MS, Graduate Student
- Marie Cronin, BA, Postbaccalaureate Intramural Research Training Award Fellow
Failure of cortical microcircuits to properly regulate excitatory/inhibitory (E/I) balance is a key feature in the etiology of several developmental psychiatric disorders and neurological diseases, such as schizophrenia, autism, ADHD, and epilepsy. E/I balance is important to synchronize the firing pattern of local neuron ensembles, and its dysregulation can degrade cognitive functions and, in extreme cases, result in epileptiform activity. Network activity, in particular oscillatory activity in the gamma-frequency range (30–80 Hz), is altered in many psychiatric disorders and may account for their cognitive and behavioral symptoms. We are interested in how Neuregulin and its receptor ErbB4, which are both genetically linked to psychiatric disorders, function in an activity-dependent fashion (i.e., experience) in the developing rodent brain to regulate synaptic plasticity, neuronal network activity, and behaviors that model features of many psychiatric disorders. We identified functional interactions between the Neuregulin/ErbB4, dopaminergic, and glutamatergic signaling pathways in GABAergic interneurons that are critical for understanding how Neuregulins regulate neuronal plasticity, E/I balance, and synchronous activity in neuronal networks.
Our earlier studies demonstrated that, in the hippocampus and neocortex, expression of ErbB4, the major Neuregulin neuronal receptor, is restricted to GABAergic interneurons. ErbB4 levels are especially high in a subtype of GABAergic neurons known as parvalbumin-positive (Pv+) fast-spiking interneurons, which are necessary for the generation and modulation of gamma oscillations. Using genetically targeted mouse mutant models (i.e., knockout [KO] mice), we went on to show that Neuregulin–ErbB4 signaling regulates synaptic plasticity, neuronal network activity (i.e., gamma oscillations), and behaviors associated with psychiatric disorders. ErbB4 receptors are also expressed prominently in dopaminergic neurons. In the recent studies described below, we generated mouse KO models that delete ErbB4 selectively in dopamine (DA)–producing neurons and showed that the mice exhibit a pronounced imbalance of DA levels and altered cognitive behaviors. More recently, our group also began to shed light on how the structure of different unprocessed Neuregulin ligands (i.e. proNRGs) determines their trafficking to distinct neuronal subcellular compartments, their post-translational processing in response to neuronal activity, and their functions in the developing and maturing nervous system.
In order to study the regulation of Neuregulin-ErbB4 signaling and its functional significance during neural development, our laboratory uses a combination of technical approaches, including: electrophysiological recordings of dissociated neurons or in acute brain slices prepared from normal and genetically altered mice; multi-electrode field recordings from brains of freely moving rats; reverse-microdialysis neurochemistry; confocal fluorescence microscopy in fixed and live tissue; fluorescence in situ hybridization using RNAscope and BaseScope; protein biochemistry including proteomics analyses; and behavioral testing. The ultimate goal of this multi-disciplinary approach is to generate holistic models to investigate the developmental impact of genes that modulate E/I balance and neuronal network activity and that consequently affect behaviors and cognitive functions that are altered in many psychiatric disorders.
Subcellular distribution and functions of single transmembrane (TM) Neuregulins in central neurons
Brain-expressed Neuregulins (NRGs), ligands for the ErbB family of receptor tyrosine kinases (ErbB2-ErbB4), originate from three different genes (NRG1-NRG3) and their diversity is greatly increased by the use of distinct promoters (NRG1: type -I, -II and -III) and alternative splicing, which generates numerous isoforms. While NRG complexity has been known for decades, the functional significance of this evolutionarily conserved diversity remained poorly understood. Recently, we made a series of discoveries that have begun to uncover how NRGs can be assigned to different classes of ligands based on their single (NRG1 type-I and -II, NRG2) or dual (NRG1 type-III, NRG3) trans-membrane (TM) topologies. First, we demonstrated that the single vs. dual TM topologies impart different subcellular trafficking properties to the unprocessed Neuregulin proforms (proNRGs). Single TM proNRGs are targeted to the cell body plasma membrane and accumulate at contact sites between the endoplasmic reticulum and overlying plasma membrane (Figure 1A), in a specialized site known as subsurface cisternae (SSCs). Unexpectedly, we found no evidence of single TM NRGs in axons; instead, proNRGs accumulate at SSCs as large somato-dendritic puncta that are most prominent in CNS GABAergic interneurons or at C-boutons on spinal cord/medulla cholinergic neurons. In response to neural activity, specifically requiring activation of N-methyl-d-aspartate glutamate receptors (NMDARs), we observe that single TM proNRGs disperse from SSCs and become susceptible to proteolytic processing by extracellular alpha secretases. Importantly, proNRGs processing results in the release of the biologically active NRG1 (type-I or -II) or NRG2 ectodomains into the extracellular space (Figure 1B). We went on to demonstrate that the NRG2 ectodomain binds to ErbB4 on GABAergic interneurons, promotes signaling and association with NMDARs, and results in the internalization of NMDARs. Taken together, our work has uncovered a bidirectional signaling pathway between NRG/ErbB4 and NMDARs that can function as a homeostatic mechanism to regulate interneuron excitability [Reference 1].
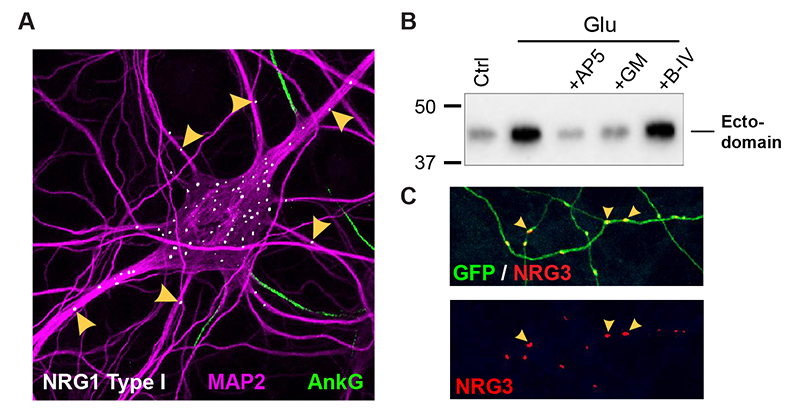
Click image to view.
Figure 1. Differential trafficking of NRG isoforms in transfected hippocampal neurons
A. NRG1 type I accumulates as puncta on soma and proximal MAP2–positive dendrites, while axons (proximal segments identified by Ankyrin G) and more distal dendrites are negative.
B. Stimulation of neurons with 20 μM glutamate (Glu) promotes NRG1 ectodomain shedding. The effect is blocked by NMDA receptor (AP5) or metalloprotease (GM6001) inhibition.
C. NRG3 puncta accumulate on GFP–filled axons, away from cell bodies.
Subcellular distribution of dual transmembrane Neuregulins in central neurons
In contrast to single TM NRGs, the dual TM NRG1-CRD (type-III) is targeted to axons where it signals in juxtacrine mode. Unexpectedly, we recently found that NRG3 is also a dual TM protein and, like NRG1-CRD (cysteine-rich domain), is targeted to axons (Figure 1C). Unlike single TM NRGs, dual TM NRGs are processed constitutively by beta-secretase (BACE), and cleavage occurs independently of NMDAR activity. Interestingly, processing is a prerequisite for axonal accumulation of dual TM NRGs; treatments that interfere with processing cause intracellular accumulation of their pro-forms [Reference 1]. Taken together, our recent findings concerning the relationships between transmembrane topology, modes of proteolytic processing, subcellular distribution, and signaling modality suggest that single and dual TM NRGs regulate neuronal functions in fundamentally different ways (Figure 2). The work was supported by a Directors Investigator Award [Reference 1].
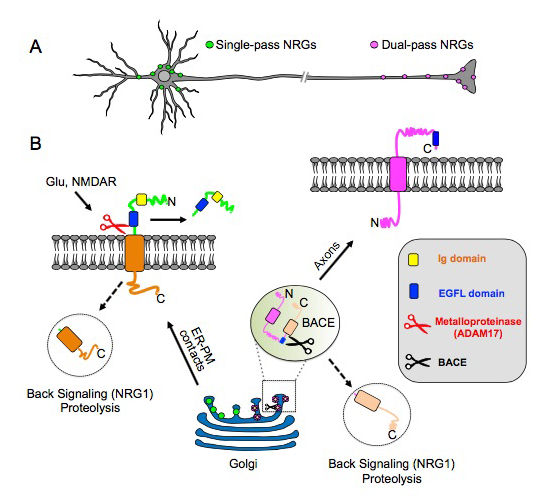
Click image to view.
Figure 2. Transmembrane (TM) topology of unprocessed pro–NRGs determines their neuronal subcellular targeting and processing in central neurons.
A. A schematic presentation, based on our findings [Reference 1], that depicts single TM NRGs accumulating at somatic subsurface cistern and at proximal dendrites to mediate NRG\ErbB4 signaling, whereas dual TM NRGs accumulate on axons and their terminals to drive long-range signaling.
B. Single TM NRGs are trafficked through the secretory pathway as unprocessed pro-forms and accumulate at subsurface cisternae (SSC) sites, where NMDAR activity promotes ectodomain shedding. By contrast, unprocessed dual TM NRGs traffic via the Golgi and are cleaved by BACE (beta-secretase) to release the amino-terminal region, which is transported into axons in an anterograde manner. Both single– and dual–TM NRGs are processed by gamma-secretase to release carboxyl-terminal proteolytic fragments, which can “back-signal” into the nucleus to regulate transcription.
A novel ultrasensitive in situ hybridization approach to detect short sequences and splice variants with cellular resolution
Detection of short isoform-specific sequences requires RNA isolation for PCR analysis—an approach that does not register the regional and cell-type-specific distribution of isoforms. The ability to distinguish the differential expression of RNA variants in tissue is critical because alterations in mRNA splicing and editing, as well as the coding of single nucleotide polymorphisms, have been associated with numerous cancers and neurological and psychiatric disorders. Recently, we reported a novel highly specific and sensitive single-probe colorimetric/fluorescent in situ hybridization (ISH) approach, called BaseScope, that targets short exon/exon RNA splice junctions using single-pair oligonucleotide probes (50 bp). We used this approach to investigate, with single-cell resolution, the expression of four ErbB4–encoding transcripts that differ by alternative splicing of exons encoding two juxtamembrane (JMa/JMb) and two cytoplasmic (CYT–1/CYT-2) domains. First, by comparing ErbB4 hybridization on sections from wild-type and ErbB4 knockout mice (missing exon 2), we demonstrated that single-pair probes have the specificity and sensitivity to visualize and quantify the differential expression of ErbB4 isoforms (Figures 3A–F). Next, we demonstrated that expression of ErbB4 isoforms differs between neurons and oligodendrocytes (Figures 3G–J), and that the differential expression of ErbB4 isoforms is evolutionarily conserved to humans. BaseScope could serve as an invaluable diagnostic tool to detect alternative spliced isoforms, and potentially single base polymorphisms, associated with disease [Reference 2].

Click image to view.
Figure 3. Oligodendrocytes and neurons express distinct ErbB4 JM (juxtamembrane) transcripts.
The sensitivity and specificity of single-pair probe in situ hybridization was demonstrated by the presence of signals in sparse GABAergic hippocampal neurons of wild-type (WT) mice hybridized with a probe corresponding to exon 2 of the ErbB4 gene (A–C), and by the absence of signal in sections prepared from ErbB4 knockout mice that lack exon 2 (D–F). Oligodendrocyte precursors cells (OPCs) and mature oligodendrocytes express ErbB4 JMa isoforms, which are susceptible to shedding and back-signaling (G,H), whereas GABAergic neurons express the cleavage-resistant JMb ErbB4 receptor (I,J).
Neuregulin-2 knockout mice exhibit dopamine dysregulation and severe behavioral phenotypes with relevance to psychiatric disorders.
Using RNAscope and novel antibodies generated in our lab, we found that NRG2 expression in the adult rodent brain does not overlap with that of NRG1 and is more extensive than originally reported. In agreement with earlier reports, we observed that NRG2 transcripts are expressed in granule cells of the cerebellum and dentate gyrus. In addition, we found that NRG2 transcripts and protein are expressed in the medial prefrontal cortex (mPFC) and striatum (Figure 4A). We generated NRG2 knockout mice (KO) to investigate the function of this single TM NRG in the developing brain. We found that NRG2 KO mice have higher extracellular dopamine levels in the dorsal striatum, but lower levels in the mPFC—a pattern that is similar to that reported for the imbalance of dopamine in schizophrenia patients. Like ErbB4 KO mice, NRG2 KOs performed abnormally in a battery of behavioral tasks relevant to psychiatric disorders. NRG2 KOs exhibit hyperactivity in a novelty-induced open field, deficits in prepulse inhibition, hypersensitivity to amphetamine, antisocial behaviors, reduced anxiety-like behavior in the elevated plus maze, and deficits in the T-maze alteration reward test, a task dependent on hippocampal and mPFC function. Acute administration of the antipsychotic clozapine rapidly raised extracellular dopamine levels in the mPFC and improved alternation T-maze performance (Figure 4B). Similar to mice treated chronically with NMDAR antagonists, we demonstrate that NMDAR synaptic currents in NRG2 KOs are augmented at hippocampal glutamatergic synapses and are more sensitive to ifenprodil, indicating an increased contribution of GluN2B–containing NMDARs. Our findings reveal a novel role for NRG2 in the modulation of behaviors with relevance to psychiatric disorders [Reference 3].
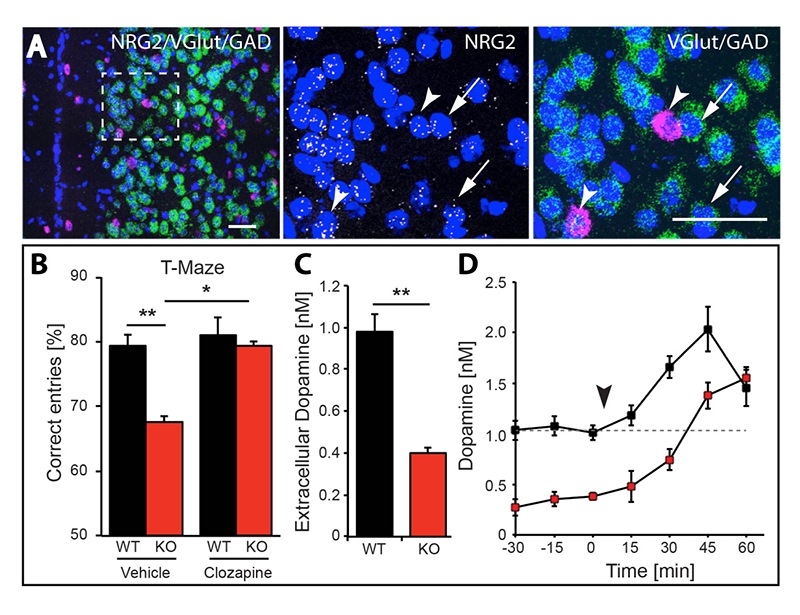
Click image to view.
Figure 4. NRG2 null mice exhibit working memory deficits and reduced dopamine levels, which are restored by clozapine.
A. Expression of NRG2 in the prefontal cortex (PFC) analyzed by triple in situ hybridization. NRG2 transcripts (white) are expressed in both glutamatergic (green) and GABAergic (magenta) neurons.
B. Poor performance by NRG2 knockout (KO) mice in a T-maze reward alternation task, as compared with WT littermates (left), can be restored by administration of the antipsychotic clozapine (right).
C. Reduced extracellular dopamine levels in the mPFC of NRG2 KO mice.
D. Extracellular dopamine levels in the mPFC of NRG2 KO mice rise after clozapine injection (arrowhead) at a time that coincides with improved performance on the T-maze.
Analysis of ErbB4 function in mice harboring targeted mutations in GABAergic and dopaminergic neurons
Dysfunctional NRG–ErbB4 signaling in the hippocampus, pre-frontal cortex (PFC), and striatum may contribute to alterations in dopamine (DA) function associated with several schizophrenia symptoms. Given that NRG1 acutely increases extracellular DA levels and regulates long-term potentiation (LTP) and gamma oscillations, and ErbB4 is expressed in GABAergic (Pv+) and mesocortical DAergic (TH+) neurons, we used genetic, biochemical, and behavioral approaches to measure DA function in the hippocampus, PFC, and striatum in mice harboring targeted mutations of ErbB4 in either PV+ or TH+ neurons. Unexpectedly, we found that, in contrast to GABAergic neurons, ErbB4 is expressed DA neuron axons and that NRG regulates extracellular DA levels by modulating dopamine transporter (DAT) function. In contrast to mice harboring mutations in GABAergic neurons, which show sensory-motor gating deficits and increases in motor activity, ErbB4 TH KO mice exhibit deficits in cognitive-related tasks (in the T-, Y- and Barnes-mazes). Therefore, direct effects of NRG/ErbB4 signaling in GABAergic vs. DAergic neurons differentially affect cortical circuits and DA homeostasis and behaviors relevant to schizophrenia [Reference 4]. Using mice that lack ErbB4 in all tissues, we are presently extending our research to the functional role of the receptor in modulating the levels of neurotransmitters and associated behaviors. Our preliminary results indicate that mice lacking ErbB4 exhibit an imbalance in extracellular dopamine levels in several brain regions, as well as behavioral deficits that resemble those observed in mice that harbor targeted mutations in either GABAergic or dopaminergic neurons.
Effects of ketamine on cortical gamma oscillations and the role of dopamine receptors in behaviors
Mounting evidence suggests that gamma oscillations are atypically high at baseline in disorders that affect attention, such as schizophrenia and ADHD. Ketamine, an antagonist of the NMDAR, has profound effects on gamma oscillation power and phenocopies schizophrenia by eliciting psychoto-mimetic symptoms and affecting cognitive functions in healthy individuals. In collaboration with Judith Walters’s lab, we used multi-electrode recordings from the medial prefrontal cortex (mPFC) and mediodorsal thalamus (MD) of rats acutely treated with ketamine, which serves as a rodent model with “face validity” for schizophrenia, to study the drug’s effects on spiking and gamma local field potentials in the mPFC and MD of freely moving rats. We found that ketamine raises gamma local field potentials and frequencies in both brain areas, but does not increase thalamocortical synchronization. Based on our prior in vitro studies, showing that a “cross-talk” between the dopamine D4 receptor (D4R) and ErbB4 regulates gamma oscillation power in acute hippocampal slices, we investigated whether and how D4R–targeting drugs regulate gamma oscillations. We found that a D4R agonist (A-412997) increased ketamine-induced gamma power, which was blocked by a D4R–selective antagonist (L-745870) in both mPFC and MD, but that neither drug altered ketamine-induced gamma power or frequency in the mPFC (Figure 5). Interestingly, in the MD, the D4R agonist increased the power of ketamine-induced gamma oscillations [Reference 5]. Experiments are in progress to evaluate the effect of mutating D4R, in conjunction with pharmacological targeting of D4R receptors, on behaviors that pertain to deficits reported in patients with schizophrenia. We are using the 5-Choice Serial Reaction Time Task (5CSRTT) to quantify the effect of the dopamine receptors on measures of attention and impulsivity.
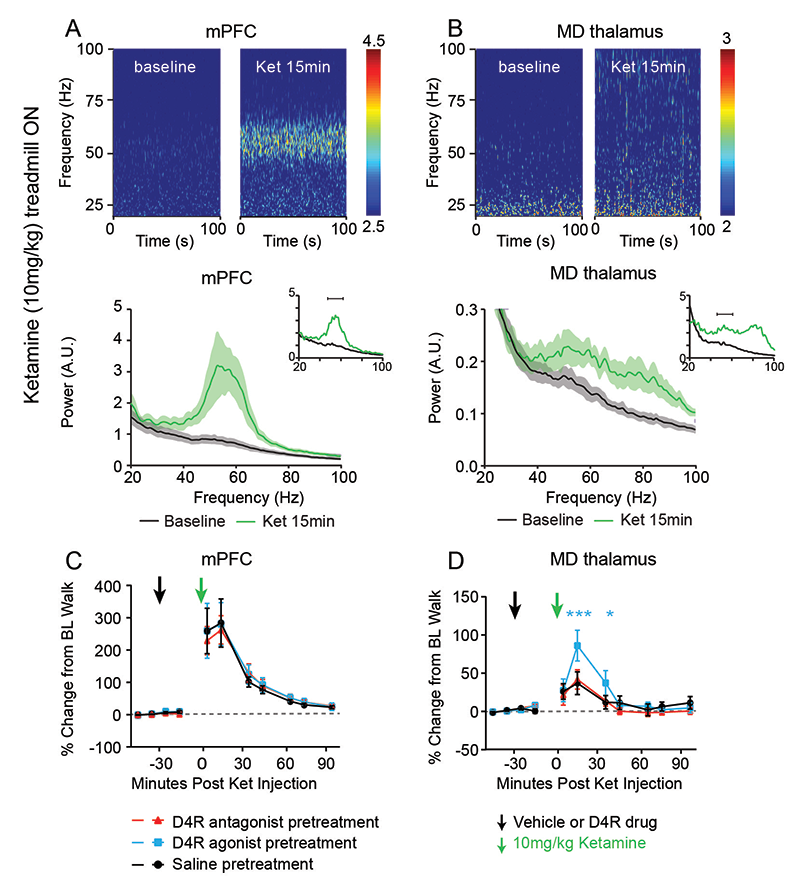
Click image to view.
Figure 5. Ketamine-induced gamma oscillations in the medial prefrontal cortex (mPFC) and medial thalamus (MD) of walking rats, and regulation by D4R agonist
Multi-electrode recordings of local field potentials in the (A) mPFC and (B) MD reveal a dramatic increase in gamma frequency (40–70Hz) power in walking rats following ketamine injection (green), compared with baseline walking by rats injected with saline (black).
C,D. Effects of D4R drugs on ketamine-induced gamma power. Administration of saline or a D4R antagonist (red) had no effect on ketamine-induced gamma power in either the (C) mPFC or the (D) MD, whereas the D4R agonist A-412997 (cyan) selectively increased gamma power in the MD.
Additional Funding
- Bench-to-Bedside Award (NHD15003-001-00001) “Selective Dopamine D4 Receptor-Targeting Compounds as Pro-Cognitive Drugs”
- NICHD DIR Director's Investigator Award (RRC# D-14-07). PI: Andres Buonanno; Co-PI: Juan Bonifacino. “Exploring the functional role of Neuregulin isoform diversity in CNS”
Publications
- Vullhorst D, Ahmad T, Karavanova I, Keating C, Buonanno A. Structural similarities between Neuregulin 1-3 isoforms determine their subcellular distribution and signaling mode in central neurons. J Neurosci 2017;37:5232-5249.
- Erben L, He MX, Laeremans A, Park E, Buonanno A. A novel ultrasensitive in situ hybridization approach to detect short sequences and splice variants with cellular resolution. Mol Neurobiol 2018;55:6169-6181.
- Yan L, Shamir A, Skirzewski M, Leiva-Salcedo E, Kwon OB, Karavanova I, Paredes D, Malkesman O, Bailey KR, Vullhorst D, Crawley JN, Buonanno A. Neuregulin-2 ablation results in dopamine dysregulation and severe behavioral phenotypes relevant to psychiatric disorders. Mol Psychiatry 2018;23:1233-1243.
- Skirzewski M, Karavanova I, Shamir A, Erben L, Garcia-Olivares J, Shin JH, Vullhorst D, Alvarez VA, Amara SG, Buonanno A. ErbB4 signaling in dopaminergic axonal projections increases extracellular dopamine levels and regulates spatial/working memory behaviors. Mol Psychiatry 2017;23:2227-2237.
- Furth KE, McCoy AJ, Dodge C, Walters JR, Buonanno A, Delaville C. Neuronal correlates of ketamine and walking induced gamma oscillations in the medial prefrontal cortex and mediodorsal thalamus. PLoS One 2017;12(11):e0186732.
Collaborators
- Veronica Alvarez, PhD, Section on Neuronal Structure, NIAAA, Rockville, MD
- Susan G. Amara, PhD, Laboratory of Molecular and Cellular Neurobiology, NIMH, Bethesda, MD
- Jung Hwa (Susan) Cheng, PhD, EM Facility, NINDS, Bethesda, MD
- Katrina Furth, PhD, Experimental Therapeutics Branch, NINDS, Bethesda, MD
- Jennie Garcia-Olivares, PhD, Laboratory of Molecular and Cellular Neurobiology, NIMH, Bethesda, MD
- Idit Golani, MD, Technion–Israel Institute of Technology, Haifa, Israel
- Mario A. Penzo, PhD, Unit on the Neurobiology of Affective Memory, NIMH, Bethesda, MD
- Alon Shamir, PhD, Mazra Mental Health Center, Akko, Israel
- Jung-Hoon Shin, PhD, Section on Neuronal Structure, NIAAA, Rockville, MD
- Judith R. Walters, PhD, Experimental Therapeutics Branch, NINDS, Bethesda, MD
Contact
For more information, email buonanno@mail.nih.gov or visit http://smn.nichd.nih.gov.