Modeling the Biophysics of the Membrane
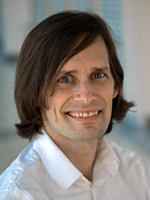
- Alexander J. Sodt, PhD, Head, Unit on Membrane Chemical Physics
- Victor Chernomordik, PhD, Volunteer
- Andrew Beaven, PhD, Postdoctoral Fellow
- Kayla Sapp, PhD, Postdoctoral Fellow
- Mitchell Dorrell, BS, Predoctoral Fellow
- Christina M. Freeman, BS, Postbaccalaureate Fellow
- Ruchita Kothari, Summer Student
- Gudrun Thorkelsdottir, Summer Student
The integrity of lipid membranes is essential for life. They provide spatial separation of the chemical contents of the cell and thus make possible the electrical and chemical potential differences that are used to transmit signals and perform work. However, the membrane must be broken frequently to form, for example, new membrane structures in the cell. The simplest structure is a vesicle to transport cargo. Such vesicles are constantly cycled between organelles and the outer plasma membrane. Thus, there is a careful balance between boundary-establishing membrane fidelity and the necessary ability of the cell to change these boundaries.
The challenge in studying the membrane is its complexity. The membrane is a thin sheet of small molecules, i.e., lipids. There are hundreds of types of lipids in the cell. Each lipid changes the properties of the membrane in its vicinity, sometimes making the sheet stiffer, sometimes softer, and sometimes acting to bend the membrane into a ball or tube. Furthermore, the lipids are constantly jostling and tangling with both each other and with proteins embedded in the membrane. To predict of how membranes are reshaped thus requires not only knowing how lipids affect the properties of the membrane surface, but also the location of specific lipids.
The question as to how molecular scale features influence big biological processes must be answered in the language of physical laws. Physics is the language of mechanism at the molecular scale. The challenge is linking these physics to "big" processes that happen in life. The technique our Unit applies is to use detailed physics-driven molecular simulation to "build up" models that can be applied at the much larger level of the cell, which requires retaining important information and eliminating irrelevant details. The software our lab develops is based around the models that we are building. Thus, a broad objective of our research is to create a publicly available software package that can be used either as a stand-alone application for analyzing membrane-reshaping processes or as a library for cellular-scale modeling packages for which the role of the membrane may be unclear or unanticipated.
Another key component of our research is to seek the best possible validation of our models. Few techniques are able to yield molecular information about lipids. Recent breakthroughs that break the diffraction-limit barrier are typically only applicable to static structures much larger than a molecular dye. In contrast, lipids are small and dynamic. We briefly discuss below our breakthroughs for comparing neutron scattering with our molecular simulations of complex lipid bilayers.
The projects use the NIH Biowulf computing cluster to run simulations and models. We use molecular dynamics software (such as NAMD and CHARMM) to conduct molecular simulations. In-house software development for eventual public distribution is a key element of the lab's work.
Modeling the response of protein structure to lateral stress
The properties of a biological membrane are determined by all components present. The outer bacterial membrane is highly enriched in "beta barrel" proteins, so-called because they are roughly cylindrical and consist of beta sheets, one of the two main forms of secondary structure found in proteins. In a computational study, we determined the response of the beta barrel proteins to lateral stress, reported as an elastic coefficient. In addition, we determined the molecular mechanism for how the protein responds to stress, in terms of the molecular features (sheet-to-sheet hydrogen bonding) that are stressed. We reported a method for determining the elastic response of membrane-embedded biomolecules to stress [Reference 1]. The results are key to determining the response of a cell or bacterium to external forces.
Methodology to compare molecular simulations of bilayers to small-angle neutron scattering (SANS) experiments
Neutron scattering can be applied to determine the molecular details of a complex structure like those of the cell. Neutrons effectively "bounce off" nuclei, creating an interference pattern at a detector, a process that is inherently quantum-mechanical. Unlike crystallography, which takes advantage of repeated elements to generate precise positional information, the information from a scattering experiment is "smeared out," representing the dynamic nature of the lipids' movement. We developed a technique to predict this "smeared out" information from molecular simulations, permitting the validation of the structure of complex membranes predicted by computational application of physical laws.
Comparison with scattering data generated by our colleagues at Oak Ridge National Laboratory indicates that our modeled structure of a complex ordered phase of lipid bilayers is an excellent match, which validates our observation that saturated lipids, when supported by cholesterol, cluster at the nanometer scale. The structure supports the hypothesis that specific lipid-lipid interactions are sufficient to drive changes in protein function in the cell.
Publications
- Lessen H, Fleming P, Fleming K, Sodt A. Building blocks of the outer membrane: calculating a general elastic energy model for ß-barrel membrane proteins. J Chem Theory Comput 2018;14:4487-4497.
- Chlanda P, Mekhedov E, Waters H, Sodt A, Schwartz C, Nair V, Blank PS, Zimmerberg J. Palmitoylation contributes to membrane curvature in Influenza A virus assembly and hemagglutinin-mediated membrane fusion. J Virol 2017;91:e00947-17.
Collaborators
- David Allender, PhD, Kent State University, Kent State, OH
- Olaf Sparre Andersen, MD, Weill Medical College of Cornell University, New York, NY
- Karen G. Fleming, PhD, The Johns Hopkins University, Baltimore, MD
- Frederick Heberle, PhD, Oak Ridge National Lab, Oak Ridge, TN
- Margaret Johnson, PhD, The Johns Hopkins University, Baltimore, MD
- John Katsaras, PhD, Oak Ridge National Laboratory, Oak Ridge, TN
- Edward Lyman, PhD, University of Delaware, Newark, DE
- Michael Schick, PhD, University of Washington in St. Louis, St. Louis, MO
Contact
For more information, email alexander.sodt@nih.gov or visit http://sodtlab.nichd.nih.gov.